Abstract
Asthma represents a chronic inflammatory airway disease with a steadily increasing global prevalence in recent decades. Animal models have proven invaluable in elucidating the underlying disease mechanisms and identifying innovative therapeutic approaches. The murine model is extensively used to investigate key characteristics of allergic asthma, including airway inflammation, airway hyperresponsiveness (AHR), and airway remodeling. Classic protocols involving sensitizing and challenging animals with different types of allergens and modes of administration are major factors in inducing asthmatic features in a mouse model. The present review critically analyzes the commonly used sensitization and allergen challenge protocols for inducing acute and chronic inflammation in the airways of mouse models of asthma, emphasizing their potential in advancing therapeutic development for allergic asthma studies.
Introduction
Asthma affects approximately 300 million people globally and continues to exhibit a rising trend every year1. Its intricate nature arises from a complex interplay of genetic and environmental factors2. Allergic asthma, the typical phenotype in clinical asthma, is triggered by allergen exposure, manifesting as a chronic inflammatory disorder affecting the airways. Key features of asthma include airway inflammation, eosinophilia, goblet cell hypersecretion, airway hyperresponsiveness (AHR), and airway remodeling3.
The cellular and biochemical processes underlying the development of allergic airways, associated with airway inflammation and remodeling, have been investigated in clinical and animal studies4. Studying asthma in humans is ethically challenging, although it is the best approach to understand the pathophysiology of the disease and to investigate drug efficacy for new drug development in allergic asthma. Hence, the utilization of animal models is essential for a comprehensive understanding of the disease, notwithstanding their limitations in replicating the complexity of human asthma.
The mouse model is widely employed to investigate the involvement of various cells and mediators, as well as structural and physiological manifestations of allergic asthma progression. This review focuses on the establishment of allergic asthma, incorporating different types of allergens and administration methods during sensitization and challenge in an asthmatic mouse model.
Allergic-induced type 2 eosinophilic asthma
In general, asthma is categorized into type 2 and non-type 2 inflammation based on distinct endotypes (Figure 1). Airway inflammation in type 2 immune response-driven asthma is phenotypically expressed as eosinophilic asthma, while non-type 2 immune response-driven asthma is characterized as neutrophilic asthma and paucigranulocytic asthma5.
Eosinophilic asthma is marked by increased eosinophil production and infiltration in the airways in response to an allergen. In type 2 immune response-driven asthma, the increase in T helper 2 (Th2) lymphocytes in the peripheral blood of asthmatic patients during an exacerbation is related to the severity of airway eosinophilia, contributing to the pathophysiological changes that require aggressive treatment6. Upon contact with allergens presented by antigen-presenting cells (APCs) in the airway, Th2 cells secrete Th2 cytokines such as interleukin (IL)-4, IL-5, and IL-13, which recruit inflammatory cells (including eosinophils, basophils, and mast cells) and activate B cells to release immunoglobulin E (IgE) (Figure 2)7.
IL-13 targets goblet cells, leading to excessive mucus production and goblet cell hyperplasia; it also induces eosinophil infiltration by priming the vessel wall, resulting in AHR8. IL-5 participates in the development, activation, and migration of eosinophils from the bone marrow to the airways, initiating airway inflammation9. IL-4 initiates IgE isotype class switching in B cells and upregulates the IgE receptor (FcεRI) on the mast cell surface, resulting in the release of histamine and other mediators3. Another hallmark of asthma is the elevated level of serum IgE synthesized by plasma cells activated by IL-4-induced class switching of B cells10.
Mouse strain in the allergic asthma mouse model
Mouse strains exhibit diverse capabilities in manifesting specific diseases and play a major role in ensuring the successful development of intended phenotypes. The most widely preferred strains include BALB/c, C57BL/6, and A/J mice11.
The BALB/c strain has become particularly prominent in asthma studies involving allergen challenge due to its proficiency in activating a robust type 2 immune response12. This includes the production of Th2 cytokines, allergen-specific IgE, eosinophilic responses, and AHR. Upon allergen exposure, BALB/c mice readily produce these Th2 cytokines and develop AHR and airway inflammation, characterized by eosinophilic infiltration—all of which are crucial to the pathogenesis of allergic asthma13, 14. Furthermore, their strong tendency to produce IgE antibodies in response to allergens facilitates the sensitization phase of allergic asthma. Upon allergen re-exposure, the crosslinking of IgE to mast cells subsequently triggers degranulation and the release of inflammatory mediators15, 16. Moreover, BALB/c mice exhibit airway remodeling, demonstrating their capability to express the pathophysiology of the inflammatory process in asthma17.
In contrast, the C57BL/6 strain is regarded as a prototypic non-type 2 mouse strain, eliciting Th1 cytokines (interferon-gamma (IFN-γ) and tumor necrosis factor alpha (TNF-α)) in response to allergen challenge18. Despite limitations in allergic airway development—particularly in IgE expression and AHR to methacholine—this strain is widely employed as a genetically modified animal model for assessing the impact of genetic manipulation on disease progression, including evaluation of allergen sensitization responsiveness and allergic airway inflammation19. Researchers also utilize other strains, such as A/J, in mouse models of asthma, demonstrating effectiveness in inducing AHR and increasing cytokine production20.
Nevertheless, mouse models of asthma do not perfectly recapitulate the complexity of human asthma, largely due to the heterogeneous nature of the disease with various phenotypes. Modeling the full spectrum of human asthma in a single mouse is challenging, often necessitating a focus on specific mechanisms, such as Th2-mediated inflammation and AHR21. Significant differences exist between mouse and human airway anatomy and physiology, including variations in size, structure, and branching patterns that affect allergen delivery and the development of airway inflammation and remodeling22. While the mouse and human immune systems share similarities, genetic variations lead to significant differences, particularly in cytokine profiles, receptor expression, and gene regulation, which influence asthma development and progression23. Furthermore, artificial allergen sensitization protocols commonly used in mouse models—often involving repeated exposure to high doses of purified allergens—differ from natural human allergen exposure, which is typically more chronic and involves a complex mixture of allergens24.
Allergens used to induce asthma
An allergen is any substance recognized as foreign by the immune system, provoking an allergic response. Different types of allergens can induce asthmatic conditions in animal models, with ovalbumin (OVA) being a commonly employed allergen. Whether in acute or chronic models, OVA offers advantages such as affordability, availability, a highly purified antigen, well-defined major histocompatibility complex (MHC) epitopes, and the existence of a recombinant peptide, making it a popular choice25.
The allergic reaction induced by OVA produces a rapid, strong, and standardized response. The OVA-sensitized and challenged mouse models have successfully elucidated the effects of inflammatory cell infiltration, Th2 cytokine secretion, eosinophil recruitment, AHR, and airway remodeling26. Additionally, some studies have reported goblet cell hyperplasia, increased mucus production, collagen deposition, and fibrosis27.
While the OVA model has greatly contributed to understanding the mechanisms of allergic asthma, concerns persist regarding its clinical relevance. Challenges in using OVA-induced asthma models include the development of OVA tolerance during long-term interventions in chronic models, the discrepancy between the human (airway) and mouse (intraperitoneal) sensitization routes—which may bypass the innate airway immune environment—and the rarity of encountering OVA in human asthma28. Consequently, other models using allergens more closely related to human asthma, such as house dust mite (HDM), have been developed.
Dermatophagoides farinae (American HDM) and Dermatophagoides pteronyssinus (European HDM) are common aeroallergens known to cause allergic sensitization29. HDM inhalation triggers pattern recognition receptors (PRRs) on airway epithelial cells, leading to chemokine and cytokine secretion that cause damage to the airway epithelia30. The allergenicity of HDM depends on its allergenic protein load, reflected by the IgE-binding complex pattern measured by the antibody titer.
HDM sensitization and challenge in mouse models have successfully reproduced asthmatic features31. Immunotherapy with purified natural D. pteronyssinus reduced AHR, eosinophilia, and Th2 cytokines in mice, indicating potential clinical effects32. Der p 2.1 peptide treatment has demonstrated the ability to suppress Th2 and Th17 cell polarization via IL-10-secreting dendritic cells33. Derp2-FlaB fusion protein, used as a treatment in HDM-sensitized mice, inhibited AHR, eosinophil infiltration, and Derp2-specific IgE, suggesting promise as a vaccine in asthma therapy34.
Additionally, other allergens have also been used for sensitization and challenge in asthmatic mouse models. Acute allergic inflammation induced by papain was observed to stimulate eosinophilia9. Intratracheal challenge with Schizophyllum commune fungus in an OVA-induced model increased airway neutrophilia and the secretion of IL-17A and IL-17F35. Coal fly dust used to sensitize BALB/c mice enhanced neutrophil and other inflammatory cell infiltration, as well as increased cytokine secretion36. Sensitization and challenge in mice using shrimp tropomyosin resulted in eosinophilia, increased IgE secretion, lung inflammation, mucus hypersecretion, goblet cell hyperplasia, collagen deposition, and dense smooth muscle, indicating that shrimp tropomyosin can be employed as an allergen to study asthma pathogenesis37.
Allergen sensitization in mouse models
Sensitization procedures are essential for inducing asthmatic conditions in animal models. Since asthma does not naturally develop in mice, sensitization is necessary to introduce the allergen and requires multiple re-exposures to evoke the allergic reaction. The initial exposure to the allergen stimulates T lymphocytes to secrete Th2 cytokines, while B lymphocytes undergo isotype switching, generating allergen-specific IgE38. Subsequent re-exposures lead to the cross-linking of basophils and IgE-bound mast cells, triggering degranulation and the release of inflammatory mediators.
Allergens are commonly used to induce allergic responses in animal models, together with adjuvants to enhance the immunogenicity of the allergen and further support the development of asthmatic animal models39. OVA, HDM, and Aspergillus are clinically relevant allergens in humans and are commonly used in allergic asthma mouse models. OVA, a protein allergen mainly found in chicken’s egg white, is widely used in the majority of studies on allergic asthma40. Various routes of sensitization, including intraperitoneal (i.p.), subcutaneous (s.c.), intranasal (i.n.) injection, and epicutaneous (ec), can be used to induce asthmatic conditions.
In the development of animal allergic asthma models, an adjuvant is administered to enhance the sensitization mechanism of allergens during the sensitization phase. Aluminum hydroxide (alum), frequently used as an adjuvant, induces a strong type 2 immune reaction41. The aggregate structure of alum continuously releases antigen, promoting phagocytosis and inducing local inflammation, resulting in macrophage activation, MHC class II expression, and antigen presentation42. The recruitment of macrophages and dendritic cells was observed in the alum-adjuvant group, with increased eosinophilic infiltration, Th2 cytokines, and IgE levels43.
In contrast, Complete Freund’s Adjuvant (CFA) induces Th17 and Th1 cell activation, resulting in neutrophilic infiltration of the lungs44. A few studies have reported significant neutrophil infiltration and low eosinophil numbers, indicating that CFA is effective in inducing neutrophilic asthma45. The lungs were also dominated by dendritic cells, macrophages, and activated B cells, with increases in the Th1 cytokine IFNγ and the Th17 cytokine IL-17A43. Interestingly, different allergens administered with the same adjuvant produced different effects, where subcutaneous injection of OVA/CFA showed neutrophilic inflammation46, whereas HDM/CFA exhibited mixed eosinophilic-neutrophilic inflammation47. This difference is possibly due to the distinct nature of the antigens and how they interact with the immune system. When combined with strong adjuvants like CFA, OVA, a relatively simple protein, may preferentially stimulate a robust Th1 immune response48, whereas HDM, a complex mixture of proteins, can activate a broader immune response, engaging both Th2 and Th17 cells49.
Meanwhile, lipopolysaccharide (LPS) is widely used to induce mixed eosinophilic and neutrophilic inflammation in asthmatic mouse models50. LPS activates toll-like receptor 4 (TLR4) on lung epithelial cells, transducing a pro-inflammatory signaling pathway51. The concentration of inhaled LPS during sensitization determines the type of inflammation, where low levels of LPS lead to Th2 responses, while high levels induce Th1 responses52.
Nevertheless, the use of adjuvants can alter experimental animal behavior by causing distress and interfering with the study of adjuvant-containing drugs, such as allergen-specific immunotherapy for allergy vaccine development53. Hence, adjuvant-free sensitization offers a more realistic model, mirroring chronic asthma manifestation in humans54. Adjuvant-free sensitization via subcutaneous injection can induce AHR, airway remodeling, increased IgE secretion, and eosinophil and lymphocyte infiltration55. Likewise, the intranasal route can induce allergic inflammation associated with Th2 cytokine secretion, increased inflammatory cell infiltration, and mucus hypersecretion53.
Therefore, the route of sensitization, as well as the types of adjuvants and allergens used, play pivotal roles in inducing different phenotypes of asthma inflammation. The presence of various adjuvants in allergen sensitization leads to different inflammatory responses in the asthmatic airway (Table 1).
Allergen | Adjuvant | Strain | Route | Efficacy | Reference |
OVA | Alum | BALB/c | i.p. | ↑ eosinophils and B cells population ↓ GATA3 and ILC2s in LN ↓ IFN-γ and Th1 cells in lung ↑ IL-5 and IL-4 and Th2 cells in lung and LN | 52 |
LPS | BALB/c | i.p. | ↓ eosinophils percentage ↑ neutrophils population in BALF ↑ T-bet and ILC1s in lungs ↑ RORγt and ILC3s in LN ↑ Th17 cells in lungs and LN | ||
OVA | CFA | C57BL/6 | i.p. | ↑ neutrophils and macrophages in BALF ↑ inflammatory cells infiltration and goblet cells based on H&E and PAS staining ↑ S100A9, caspase-1, IL-1β, IL-17, IFN-γ, TNF-α and myeloperoxidase proteins in western blot analysis | 45 |
OVA | CFA | C57BL/6 | i.p. | ↑ plasmacytoid dendritic cells, exudate macrophages, and B cells ↑ neutrophils in BALF and lung ↑ Th1 cytokine IFN-γ | 43 |
Alum | C57BL/6 | i.p. | ↑ interstitial macrophages and myeloid dendritic cells ↑ eosinophils in BALF and lungs ↑ IL-5 and IL-13 ↑ basophils and mast cells in lung tissue | ||
OVA | Alum | BALB/c | i.p. | ↑ eosinophils number ↑ IL-4, IL-5, IL-13 and IL-33 in BALF Moderate inflammation (only bronchi and vessels of the lungs infiltrated with inflammatory cells) | 56 |
LPS | BALB/c | i.p. | ↑ neutrophils number ↑ Th1 (IFN-γ) and Th17 (IL-17A) in BALF Severe inflammation (nearly whole lung infiltrated with inflammatory cells) | ||
HDM | Alum | BALB/c | s.c. | ↑ IgE level ↑ Th2 cytokines | 57 |
HDM | CFA | C57BL/6 | s.c. | ↑ macrophage MIF in BALF ↑ mixed eosinophilic/neutrophilic response AHR | 47 |
OVA | CFA | BALB/c | s.c. | ↑ neutrophils count ↑ inflammatory cell infiltration AHR | 46 |
OVA | LPS | BALB/c | i.n. | ↑ Th2 (IL-4, IL-5, IL-13) and Th17 (IL-17) ↓ Th1 (IFN-γ) and Treg (TGF-β, IL-10) ↑ GATA3, T-bet, and ROR-γt expression ↓ T-bet, Foxp3 and IL-10 expression AHR | 58 |
Strain/gender | Allergen | Sensitization/route | Challenge/route | Responses to challenge | References |
BALB/c Female | OVA | Day 0 and 7 OVA + alum i.p. | Day 14-18 OVA i.n. | AHR and airway inflammation | 59 |
BALB/c Female | HDM | Day 0 and 7 HDM + alum i.p. | Day 14-25 HDM i.n. | AHR, inflammatory cells infiltration, eosinophilia, Th2 cytokines and IL-33 secretion | 60 |
BALB/c Male | OVA | Day 0 and 14 OVA + alum i.p. | Day 21-23 OVA Aerosol | Neutrophils and eosinophil infiltration airway wall thickening | 52 |
C57BL/6 Female | OVA | Day 0 and 5 OVA + alum i.p. | Day 12 and 13 OVA Aerosol | Neutrophilia and airway inflammation | 35 |
BALB/c Female | OVA | Day 0 and 14 OVA + alum i.p. | Day 28-30 OVA Aerosol | Inflammatory cells infiltration, Th2 cytokines secretion, eosinophilia | 61 |
BABL/c Male | OVA | Day 7 and 14 OVA + alum i.p. | Day 21-23 OVA Aerosol | Leukocytes infiltration, eosinophilia and TNF-α, IL-1β, IL-6, TGF-β, and IFN-γ secretion | 62 |
Balb/c Male | OVA | Day 0, 2, 4, 7, 9 and 10 OVA i.p. | Day 15, 18 and 21 OVA i.t. | Inflammatory cells infiltration, muscle and epithelial thickening, epithelial desquamation, goblet cell metaplasia, and collagen deposition | 17 |
BALB/c Female | OVA | Day 1 and 14: OVA + alum i.p. | Day 25-28 OVA i.n. | Inflammatory cells inflammation and IL-5 and IL-13 secretion | 63 |
C57BL/6 Female | OVA | Day 1 and 15 OVA + alum i.p. | Day 21-23 OVA Aerosol | Aberrant miRNAs profile in the CD4 + T lymphocytes | 64 |
BALB/c Female | OVA | Day 1, 8 and 15 OVA + alum i.p. | Day 16-22 OVA Aerosol | Airway inflammation and remodeling, inflammatory cells infiltration and Th2 cytokines secretion | 65 |
Allergen challenge in mouse models
The capability of mouse models to induce the asthmatic condition is well-established, and these models are useful for controlling inflammation. The acute allergic airway inflammatory model is predominantly studied due to its ability to successfully establish many asthmatic features. However, this acute model falls short in developing other major features observed in human asthma, such as collagen deposition and chronic airway remodeling. Consequently, the field has shifted toward developing and studying chronic allergic airway inflammation models to address the limitations of the acute model.
Acute allergen challenge model
Because mice do not naturally develop asthma, human intervention is necessary to induce artificial asthmatic conditions in the airways. Asthma is characterized by multiple phenotypes and cannot be entirely replicated by a single model. Hence, specific phenotypes are developed depending on the objectives of the study. Table 2 provides a summary of different sensitization and challenge protocols in acute asthmatic mouse models.
The development of an asthmatic model in mice depends on several factors, including the protocol of sensitization and challenges, the adjuvants, and the type of allergens. In the acute mouse asthma model, diverse yet coherent protocols were employed. Allergen sensitization via systemic delivery into the circulatory system commonly necessitates multiple re-exposures to establish a favorable allergic model38. Meanwhile, allergen challenge is usually administered via the airways through inhalation (aerosol), intratracheal (i.t.), or intranasal (i.n.) routes. The common acute model protocol involves allergen sensitization lasting for two to three weeks, followed by allergen challenge for several consecutive days, with the endpoint assessed 24 hours after the last challenge.
The acute mouse model develops the common characteristics of clinical asthma. Studies have shown that lung pathology induced by allergens can exhibit changes in the lungs that cause airway inflammation, airway remodeling, and AHR66, 67. Histological analysis allows examination of inflammatory cell recruitment, mucus production, collagen deposition, and fibrosis in the perivascular and peribronchiolar space68. The acute model is also utilized to study the mechanisms of remodeling and oxidative stress associated with the signaling pathway in pulmonary asthma69, 70. Additionally, this model has also shown the amelioration of allergic inflammation when treated with various potential suppressors, such as IL-3871, anti-IL-2572, and leukotriene B4 receptor blocker73.
While the acute model has successfully investigated some features of the pathophysiology of asthma, it has limitations compared to clinical asthma, which requires persistent airway inflammation to mimic asthmatic individuals. The short period of allergen challenge is one reason for minimal changes in airway remodeling, AHR, and eosinophilia, with these changes subsiding a few weeks after the last challenge. Asthma is associated with chronic disease, so some concerns arise regarding the reliability of acute mouse models in investigating disease progression and potential treatments.
Chronic allergen challenge model
A chronic mouse model with prolonged allergen challenges overcomes several issues encountered in the acute mouse model. Significant differences in AHR, airway remodeling, and inflammatory profiles between acute and chronic asthmatic models have been observed in clinical asthma. The chronicity of allergen exposure is a critical concern in the acute model, as the sensitization and challenge procedures may not induce persistent changes in airway inflammation, unlike in humans. Various chronic sensitization and challenge protocols have been employed, with some summarized in Table 3.
Chronic allergen challenge contributes to persistent airway remodeling, depicted by collagen deposition, airway inflammation, goblet cell hyperplasia, and eosinophilia in the mouse model74, 75. The chronic model typically spans 4 to 12 weeks, starting with allergen sensitization followed by repeated low-level allergen exposure. Different types of allergens have been used to simulate the chronic model, and adjuvant-free protocols have been employed to imitate the natural sensitization that occurs in humans76.
The presence of T cells is essential for an immediate response to recurrent allergen exposure77. Chronic allergen exposure has demonstrated a CD4+ and CD8+ T cell-dependent effect on airway inflammatory cell infiltration and AHR76, 78. Moreover, eosinophils play an important role in the remodeling process by altering the structure of airway nerves, inducing AHR and fibrosis, and thereby increasing allergen sensitivity in eosinophilic asthma associated with chronic allergen exposure79. Extensive research using chronic murine asthma models has explored the roles of some proteins, such as the WNT5A ligand80, microRNA-22181, and IL-3374, to understand their effects on asthma pathogenesis.
The chronic mouse model has successfully replicated key features of human asthma and is currently employed to study potential therapeutic treatments applicable at the clinical stage. Extracellular vesicles derived from human umbilical cord mesenchymal stem cells have shown therapeutic potential in the chronic asthma model, particularly in a hypoxic environment82. This study demonstrated significant attenuation of airway inflammation, represented by the depletion of inflammatory cells, eosinophils, and Th2 cytokines, and amelioration of airway remodeling, accompanied by decreases in alpha-smooth muscle actin (α-SMA), collagen type 1, and transforming growth factor-beta (TGF-β) 1 signaling pathway expression.
Additionally, this model is also used to gain a better understanding of biochemical changes within complex tissue samples of potential anti-asthmatic compounds83. Novel imaging techniques that combine the analytical approaches of focal plane array (FPA) and synchrotron Fourier-transform infrared (S-FTIR) enable the investigation of broader molecular changes surrounding the airways and identification of types of collagen deposition present in the chronic asthma model, further supporting the analysis of conventional methods.
However, several hindrances related to the chronic mouse model were identified when compared to human asthma. In humans, asthma often develops spontaneously in early life alongside immature lung development, compared to the fully developed lungs of mice at birth, necessitating artificial allergen and adjuvant sensitization84. The route, amount, and frequency of allergen exposure in controlled conditions of allergic airway mouse models differ from the natural and acquired immune responses of asthma exacerbation in humans and do not reflect patient heterogeneity85, 86.
Moreover, the extended period of inhaled antigen exposure in mice induces tolerance, described by changes in inflammatory cell profiles, airway inflammation, and AHR, limiting the opportunities to investigate the chronic model and the underlying pathways87, 88. Nevertheless, allergen tolerance provides some advantages for studying the effect of certain parameters associated with asthma for therapeutic development. Inhaled allergens may induce an inappropriate Th2-cell inflammatory response, and this adverse reaction can be obscured via the local inhalation tolerance process to restore airway homeostasis89 and regulation of free IgE90, thereby diminishing asthma symptoms.
While invaluable for research, these chronic mouse models pose significant ethical challenges. Prolonged suffering, due to repeated allergen exposure leading to chronic inflammation, AHR, and airway remodeling, can cause discomfort, breathing difficulties, and potentially pain over extended periods91. Assessing pain and distress can be challenging, as subtle behavioral changes may indicate underlying suffering but are difficult to interpret definitively92. Therefore, researchers must carefully optimize research protocols by minimizing the duration and intensity of allergen exposure, balancing the need to reduce distress with the requirement to obtain meaningful data. Animals should also be monitored regularly for signs of distress, including routine assessment of respiratory function and behavior.
Strain/gender | Allergen | Sensitization/route | Challenge/route | Response to challenge | References |
BALB/c Female | OVA | Day 0, 7, and 14 OVA + alum i.p. | Day 21-55 OVA Aerosol/i.n. | Airway remodeling, inflammatory cells infiltration, eosinophilia, increased mucus production and IL-4 and IL-13 secretion | 83 |
BALB/c Female | OVA | Day 0, 7, 14, and 21 OVA + alum s.c. | Days 33 and 35: OVA i.n. | AHR, airway inflammation, and remodeling, Th2 cytokines, TSLP, IL-33 and IL-25 secretion, goblet cell hyperplasia, increased TNF-α, and collagen deposition | 93 |
BALB/c Female | OVA | Day 0, 14, 28 and 42 OVA + alum i.p. | Day 21-42 OVA Aerosol | Airway remodeling, inflammatory cells infiltration, elevated IgE, IL-6 and IL-13 | 94 |
Balb/c Female | HDM | Days 0, 7, and 14 HDM i.p. | Day 21–28 HDM i.n. | Inflammatory cells infiltration, Th2 cytokines secretion and specific IgE production, airway wall thickening, mucosal metaplasia, collagen deposition, goblet cell hyperplasia and mucus hypersecretion. | 30 |
BALB/c Female | OVA | Day 1 and 14 OVA + alum i.p. | Day 14, 17, 21, 24, 27, 60, 69, 71, 73, 74, and 75 OVA i.n. | Inflammatory cells infiltration, Th2 cytokine, IL-17, TNF-α and high mobility group box protein 1 secretion. | 95 |
BALB/c Female | OVA | Day 1, 2 and 3 OVA + alum i.p. | Day 14, 17, 21, 24, 27, 60, 69, 71, 73, 74, and 75 OVA i.n. | Airway remodeling, inflammatory cells infiltration and Th2, Th1, IL-17 and IL-22 cytokines secretion and collagen deposition | 96 |
BALB/c Female | OVA | Day 1 and 14 OVA + alum i.p. | Day 28, 30, 32, 34, 36, 38, 40, 42 and 44 OVA Aerosol | Airway inflammation, fibrotic airway remodeling and inflammatory cells infiltration | 63 |
C57BL/6 Female | HDM | Day 1 HDM i.n. | Day 2-36: HDM i.n. | Th2-mediated eosinophilic inflammation and IL-12 and IL-6 production | 97 |
Balb/c Male | OVA | Day 0 and 14 OVA+ alum i.p. | Three times per week for 9 weeks OVA Aerosol | Day 87: AHR, inflammatory cells infiltration, eosinophilia, and mucus hypersecretion | 98 |
C57BL/6 Female | HDM | Day 0 and 7: HDM i.n. | five times per week for three weeks, rested (4–8 week) and rechallenged HDM i.n. | 24 hours after the final challenge: AHR, increased CD4 + T cells and dendritic cells | 99 |
Future perspectives and conclusion
Allergen sensitization and challenge in mouse models represent classical protocols for manifesting asthma pathophysiology. Researchers are striving to model specific disease phenotypes that accurately replicate the complex nature of human asthma. While acute allergen challenges effectively represent several hallmarks of asthma, they fall short of capturing certain features of chronic asthma. Therefore, the development of chronic allergen challenge models aims to deepen understanding of disease mechanisms and discover novel therapeutic potentials.
Allergic mouse models require active sensitization, typically introduced with adjuvants administered intraperitoneally or subcutaneously alongside the allergen. These methods are less intrusive and do not require sedation, but they may induce tolerance. As a result, models without adjuvants have been developed to induce sensitization in the airways via intranasal instillation, simulating the natural exposure of humans to airborne allergens. This model has proven effective in producing a phenotype of asthma comparable to that of the traditional adjuvant model.
In allergen challenge, aerosol and intranasal routes are likely closer to mimicking human exposure than the intratracheal approach. The allergen OVA may inadvertently induce tolerance with repeated and prolonged exposure, in contrast to HDM, which exhibits persistent airway inflammation, making it more suitable for modeling chronic asthma. Therefore, adjuvant-free models and aeroallergen exposure may be more relevant in mimicking human asthma for the development of new treatments and preventive approaches. Despite the shortcomings of both acute and chronic allergic asthma models, ongoing research aims to improve protocols to enhance our understanding of asthma at the cellular and molecular levels.
Abbreviations
α-SMA (Alpha-smooth muscle actin), AHR (Airway hyperresponsiveness), Alum (Aluminum hydroxide), APC(s) (Antigen-presenting cell(s)), BALF (Bronchoalveolar lavage fluid), CFA (Complete Freund’s Adjuvant), ec (Epicutaneous), Foxp3 (Forkhead box protein 3), FPA (Focal plane array, an imaging technique), H&E (Hematoxylin and eosin staining), HDM (House dust mite), IFN-γ (Interferon-gamma), IgE (Immunoglobulin E), IL (IL-4, IL-5, IL-13, IL-17, etc.) (Interleukin), ILC(s) (ILC1, ILC2, ILC3) (Innate lymphoid cell(s)), i.n. (Intranasal), i.p. (Intraperitoneal), i.t. (Intratracheal), LN (Lymph node), LPS (Lipopolysaccharide), MHC (Major histocompatibility complex), miRNAs (Micro ribonucleic acids), OVA (Ovalbumin), PAS (Periodic acid–Schiff staining), RORγt (Retinoic acid receptor-related orphan receptor gamma t), s.c. (Subcutaneous), S-FTIR (Synchrotron Fourier-transform infrared spectroscopy), S100A9 (S100 calcium-binding protein A9), T-bet (T-box transcription factor TBX21), TGF-β (Transforming growth factor-beta), Th (Th1, Th2, Th17) (T helper cells), TLR4 (Toll-like receptor 4), TNF-α (Tumor necrosis factor alpha), and TSLP (Thymic stromal lymphopoietin)
Acknowledgments
None.
Author’s contributions
Bushra Solehah Mohd Rosdan served as the primary author and was responsible for the initial drafting and subsequent editing of the manuscript. Nurul Asma Abdullah provided supervision, conducted critical reviews, and contributed to manuscript revisions. All authors have read and approved the final version of the manuscript.
Funding
This study was funded by Research University Grant (1001/PPSK/8012344) from Universiti Sains Malaysia.
Availability of data and materials
Not applicable.
Ethics approval and consent to participate
Not applicable.
Consent for publication
Not applicable.
Competing interests
The authors declare that they have no competing interests.
References
-
James
S.L.,
Abate
D.,
Abate
K.H.,
Abay
S.M.,
Abbafati
C.,
Abbasi
N.,
Disease
GBD 2017,
Injury Incidence
Prevalence Collaborators
Global, regional, and national incidence, prevalence, and years lived with disability for 354 diseases and injuries for 195 countries and territories, 1990-2017: a systematic analysis for the Global Burden of Disease Study 2017. Lancet.
2018;
392
(10159)
:
1789-858
.
View Article PubMed Google Scholar -
Morales
E.,
Duffy
D.,
Genetics and Gene-Environment Interactions in Childhood and Adult Onset Asthma. Frontiers in Pediatrics.
2019;
7
:
499
.
View Article PubMed Google Scholar -
Athari
S.S.,
Targeting cell signaling in allergic asthma. Signal Transduction and Targeted Therapy.
2019;
4
(1)
:
45
.
View Article PubMed Google Scholar -
Liu
Y.,
Wei
L.,
He
C.,
Chen
R.,
Meng
L.,
Lipoxin A4 inhibits ovalbumin-induced airway inflammation and airway remodeling in a mouse model of asthma. Chemico-Biological Interactions.
2021;
349
:
109660
.
View Article PubMed Google Scholar -
Svenningsen
S.,
Nair
P.,
Asthma Endotypes and an Overview of Targeted Therapy for Asthma. Frontiers in Medicine.
2017;
4
:
158
.
View Article PubMed Google Scholar -
Shrestha Palikhe
N.,
Wu
Y.,
Konrad
E.,
Gandhi
V.D.,
Rowe
B.H.,
Vliagoftis
H.,
Th2 cell markers in peripheral blood increase during an acute asthma exacerbation. Allergy.
2021;
76
(1)
:
281-90
.
View Article PubMed Google Scholar -
Sze
E.,
Bhalla
A.,
Nair
P.,
Mechanisms and therapeutic strategies for non-T2 asthma. Allergy.
2020;
75
(2)
:
311-25
.
View Article PubMed Google Scholar -
Chigbu
D.I.,
Jain
P.,
Khan
Z.K.,
Immune Mechanisms, Pathology, and Management of Allergic Ocular Diseases. In: Jain, P., Ndhlovu, L. (eds) Advanced Concepts in Human Immunology: Prospects for Disease Control. Springer, Cham. https://doi.org/10.1007/978-3-030-33946-3_4Springer International Publishing: Cham; 2020.
View Article Google Scholar -
Boberg
E.,
Johansson
K.,
Malmhäll
C.,
Calvén
J.,
Weidner
J.,
R\aadinger
M.,
Interplay Between the IL-33/ST2 Axis and Bone Marrow ILC2s in Protease Allergen-Induced IL-5-Dependent Eosinophilia. Frontiers in Immunology.
2020;
11
:
1058
.
View Article PubMed Google Scholar -
Humbert
M.,
Bousquet
J.,
Bachert
C.,
Palomares
O.,
Pfister
P.,
Kottakis
I.,
IgE-Mediated Multimorbidities in Allergic Asthma and the Potential for Omalizumab Therapy. The Journal of Allergy and Clinical Immunology. In Practice.
2019;
7
(5)
:
1418-29
.
View Article PubMed Google Scholar -
Azman
S.,
Sekar
M.,
Bonam
S.R.,
Gan
S.H.,
Wahidin
S.,
Lum
P.T.,
Traditional Medicinal Plants Conferring Protection Against Ovalbumin-Induced Asthma in Experimental Animals: A Review. Journal of Asthma and Allergy.
2021;
14
:
641-62
.
View Article PubMed Google Scholar -
Fehrenbach
H.,
Wagner
C.,
Wegmann
M.,
Airway remodeling in asthma: what really matters. Cell and Tissue Research.
2017;
367
(3)
:
551-69
.
View Article PubMed Google Scholar -
Huang
W.C.,
Wu
S.J.,
Fang
L.W.,
Lin
T.Y.,
Liou
C.J.,
Phillyrin attenuates airway inflammation and Th2 cell activities in a mouse asthma model. Food and Agricultural Immunology.
2023;
34
(1)
.
View Article Google Scholar -
Lambrecht
B.N.,
Hammad
H.,
Fahy
J.V.,
The Cytokines of Asthma. Immunity.
2019;
50
(4)
:
975-91
.
View Article PubMed Google Scholar -
Gouel-Chéron
A.,
Dejoux
A.,
Lamanna
E.,
Bruhns
P.,
Animal Models of IgE Anaphylaxis. Biology (Basel).
2023;
12
(7)
:
931
.
View Article PubMed Google Scholar -
Miranda-Waldetario
M.C.,
Curotto de Lafaille
M.A.,
Making good of a tricky start: how IgE and mast cells manage a protective sway in food allergy. Immunity.
2023;
56
(9)
:
1988-90
.
View Article PubMed Google Scholar -
Maria
L.,
Leite
B.,
Guilherme
L.,
Capriglione
A.,
Histopathologic evaluation, anesthetic protocol, and physiological parameters for an experimental Balb/c mouse model of asthma.. Lungs and Breathing.
2019;
3
(1)
:
1-6
.
View Article Google Scholar -
Cardoso
F.O.,
Zaverucha-do-Valle
T.,
Almeida-Souza
F.,
Abreu-Silva
A.L.,
Calabrese
K.D.,
Modulation of Cytokines and Extracellular Matrix Proteins Expression by Leishmania amazonensis in Susceptible and Resistant Mice. Frontiers in Microbiology.
2020;
11
:
1986
.
View Article PubMed Google Scholar -
Park
M.K.,
Park
H.K.,
Yu
H.S.,
Toll-like receptor 2 mediates Acanthamoeba-induced allergic airway inflammatory response in mice. PLoS Neglected Tropical Diseases.
2023;
17
(1)
:
e001108
.
View Article PubMed Google Scholar -
Casaro
M.B.,
Thomas
A.M.,
Mendes
E.,
Fukumori
C.,
Ribeiro
W.R.,
Oliveira
F.A.,
A probiotic has differential effects on allergic airway inflammation in A/J and C57BL/6 mice and is correlated with the gut microbiome. Microbiome.
2021;
9
(1)
:
134
.
View Article PubMed Google Scholar -
Rosenberg
H.F.,
Druey
K.M.,
Modeling asthma: Pitfalls, promises, and the road ahead. Journal of Leukocyte Biology.
2018;
104
(1)
:
41-8
.
View Article PubMed Google Scholar -
Rydell-Törmänen
K.,
Johnson
J.R.,
The Applicability of Mouse Models to the Study of Human Disease. In: Bertoncello, I. (eds) Mouse Cell Culture. Methods in Molecular Biology, vol 1940. Humana Press, New York, NY. 2019;
:
3-22
.
View Article Google Scholar -
Forbester
J.L.,
Humphreys
I.R.,
Genetic influences on viral-induced cytokine responses in the lung. Mucosal Immunology.
2021;
14
(1)
:
14-25
.
View Article PubMed Google Scholar -
Radhouani
M.,
Starkl
P.,
Adjuvant-independent airway sensitization and infection mouse models leading to allergic asthma. Frontiers in Allergy.
2024;
5
:
1423938
.
View Article PubMed Google Scholar -
Mostashari
P.,
Marsza\lek
K.,
Aliyeva
A.,
Mousavi Khaneghah
A.,
The Impact of Processing and Extraction Methods on the Allergenicity of Targeted Protein Quantification as Well as Bioactive Peptides Derived from Egg. Molecules (Basel, Switzerland).
2023;
28
(6)
:
2658
.
View Article PubMed Google Scholar -
Ponci
V.,
Silva
R.C.,
Santana
F.P.,
Grecco
S.S.,
Fortunato
C.R.,
Oliveira
M.A.,
Biseugenol Exhibited Anti-Inflammatory and Anti-Asthmatic Effects in an Asthma Mouse Model of Mixed-Granulocytic Asthma. Molecules (Basel, Switzerland).
2020;
25
(22)
:
5384
.
View Article PubMed Google Scholar -
Piao
C.H.,
Bui
T.T.,
Fan
Y.J.,
Nguyen
T.V.,
Shin
D.U.,
Song
C.H.,
In vivo and in vitro anti‑allergic and anti‑inflammatory effects of Dryopteris crassirhizoma through the modulation of the NF‑ĸB signaling pathway in an ovalbumin‑induced allergic asthma mouse model. Molecular Medicine Reports.
2020;
22
(5)
:
3597-606
.
View Article PubMed Google Scholar -
Sethi
G.S.,
Naura
A.S.,
Progressive increase in allergen concentration abrogates immune tolerance in ovalbumin-induced murine model of chronic asthma. International Immunopharmacology.
2018;
60
:
121-31
.
View Article PubMed Google Scholar -
Zhang
J.,
Chen
J.,
Robinson
C.,
Cellular and Molecular Events in the Airway Epithelium Defining the Interaction Between House Dust Mite Group 1 Allergens and Innate Defences. International Journal of Molecular Sciences.
2018;
19
(11)
:
3549
.
View Article PubMed Google Scholar -
Liu
M.,
Lu
J.,
Zhang
Q.,
Zhang
Y.,
Guo
Z.,
Clara cell 16 KDa protein mitigates house dust mite-induced airway inflammation and damage via regulating airway epithelial cell apoptosis in a manner dependent on HMGB1-mediated signaling inhibition. Molecular Medicine (Cambridge, Mass.).
2021;
27
(1)
:
11
.
View Article PubMed Google Scholar -
Park
S.J.,
Lee
K.,
Kang
M.A.,
Kim
T.H.,
Jang
H.J.,
Ryu
H.W.,
Tilianin attenuates HDM-induced allergic asthma by suppressing Th2-immune responses via downregulation of IRF4 in dendritic cells. Phytomedicine : International Journal of Phytotherapy and Phytopharmacology.
2021;
80
:
153392
.
View Article PubMed Google Scholar -
Hesse
L.,
van Ieperen
N.,
Habraken
C.,
Petersen
A.H.,
Korn
S.,
Smilda
T.,
Subcutaneous immunotherapy with purified Der p1 and 2 suppresses type 2 immunity in a murine asthma model. Allergy.
2018;
73
(4)
:
862-74
.
View Article PubMed Google Scholar -
Klein
M.,
Colas
L.,
Cheminant
M.A.,
Brosseau
C.,
Sauzeau
V.,
Magnan
A.,
Der p 2.1 Peptide Abrogates House Dust Mites-Induced Asthma Features in Mice and Humanized Mice by Inhibiting DC-Mediated T Cell Polarization. Frontiers in Immunology.
2020;
11
:
565431
.
View Article PubMed Google Scholar -
Tan
W.,
Zheng
J.H.,
Duong
T.M.,
Koh
Y.I.,
Lee
S.E.,
Rhee
J.H.,
A Fusion Protein of Derp2 Allergen and Flagellin Suppresses Experimental Allergic Asthma. Allergy, Asthma & Immunology Research.
2019;
11
(2)
:
254-66
.
View Article PubMed Google Scholar -
Hanashiro
J.,
Muraosa
Y.,
Toyotome
T.,
Hirose
K.,
Watanabe
A.,
Kamei
K.,
Schizophyllum commune induces IL-17-mediated neutrophilic airway inflammation in OVA-induced asthma model mice. Scientific Reports.
2019;
9
(1)
:
19321
.
View Article PubMed Google Scholar -
Saba
E.,
Lee
Y.S.,
Yang
W.K.,
Lee
Y.Y.,
Kim
M.,
Woo
S.M.,
Effects of a herbal formulation, KGC3P, and its individual component, nepetin, on coal fly dust-induced airway inflammation. Scientific Reports.
2020;
10
(1)
:
14036
.
View Article PubMed Google Scholar -
Fang
L.,
Zhou
F.,
Wu
F.,
Yan
Y.,
He
Z.,
Yuan
X.,
A mouse allergic asthma model induced by shrimp tropomyosin. International Immunopharmacology.
2021;
91
:
107289
.
View Article PubMed Google Scholar -
Sadeghi
M.,
Koushki
K.,
Mashayekhi
K.,
Ayati
S.H.,
Shahbaz
S. Keshavarz,
Moghadam
M.,
DC-targeted gold nanoparticles as an efficient and biocompatible carrier for modulating allergic responses in sublingual immunotherapy. International Immunopharmacology.
2020;
86
:
106690
.
View Article PubMed Google Scholar -
Raspe
J.,
Schmitz
M.S.,
Barbet
K.,
Caso
G.C.,
Cover
T.L.,
Müller
A.,
Therapeutic properties of Helicobacter pylori-derived vacuolating cytotoxin A in an animal model of chronic allergic airway disease. Respiratory Research.
2023;
24
(1)
:
178
.
View Article PubMed Google Scholar -
Sharif
M.,
Anjum
I.,
Shabbir
A.,
Syed
S. Khurram,
Mobeen
I.,
Shahid
M. Hassaan,
Amelioration of Ovalbumin-Induced Allergic Asthma by Juglans regia via Downregulation of Inflammatory Cytokines and Upregulation of Aquaporin-1 and Aquaporin-5 in Mice. Journal of Tropical Medicine.
2022;
2022
:
6530095
.
View Article PubMed Google Scholar -
Kim
C.Y.,
Kim
J.W.,
Kim
J.H.,
Jeong
J.S.,
Lim
J.O.,
Ko
J.W.,
Inner Shell of the Chestnut (Castanea crenatta) Suppresses Inflammatory Responses in Ovalbumin-Induced Allergic Asthma Mouse Model. Nutrients.
2022;
14
(10)
:
2067
.
View Article PubMed Google Scholar -
Ko
E.J.,
Lee
Y.T.,
Kim
K.H.,
Lee
Y.,
Jung
Y.J.,
Kim
M.C.,
Roles of Aluminum Hydroxide and Monophosphoryl Lipid A Adjuvants in Overcoming CD4+ T Cell Deficiency To Induce Isotype-Switched IgG Antibody Responses and Protection by T-Dependent Influenza Vaccine. The Journal of Immunology : Official Journal of the American Association of Immunologists.
2017;
198
(1)
:
279-91
.
View Article PubMed Google Scholar -
Özkan
M.,
Eskiocak
Y.C.,
Wingender
G.,
Macrophage and dendritic cell subset composition can distinguish endotypes in adjuvant-induced asthma mouse models. PLoS One.
2021;
16
(6)
:
e0250533
.
View Article PubMed Google Scholar -
Menson
K.E.,
Mank
M.M.,
Reed
L.F.,
Walton
C.J.,
Van Der Vliet
K.E.,
Ather
J.L.,
Therapeutic efficacy of IL-17A neutralization with corticosteroid treatment in a model of antigen-driven mixed-granulocytic asthma. American Journal of Physiology. Lung Cellular and Molecular Physiology.
2020;
319
(4)
:
693-709
.
View Article PubMed Google Scholar -
Lee
J.U.,
Park
J.S.,
Jun
J.A.,
Kim
M.K.,
Chang
H.S.,
Baek
D.G.,
Inhibitory Effect of Paquinimod on a Murine Model of Neutrophilic Asthma Induced by Ovalbumin with Complete Freund's Adjuvant. Canadian Respiratory Journal.
2021;
2021
:
8896108
.
View Article PubMed Google Scholar -
Zhao
M.Z.,
Li
Y.,
Han
H.Y.,
Mo
L.H.,
Yang
G.,
Liu
Z.Q.,
Specific Ag-guiding nano-vaccines attenuate neutrophil-dominant allergic asthma. Molecular Immunology.
2021;
129
:
103-11
.
View Article PubMed Google Scholar -
V. sita rama raju Allam,
I. Adcock,
K.F. Chung,
E. Morand,
J. Harris,
M. Sukkar,
MIF antagonism restores corticosteroid sensitivity in a murine model of severe asthma. European Respiratory Journal .
2018;
52
(suppl 62)
:
PA979
.
View Article Google Scholar -
Xia
M.,
Xu
F.,
Ni
H.,
Wang
Q.,
Zhang
R.,
Lou
Y.,
Neutrophil activation and NETosis are the predominant drivers of airway inflammation in an OVA/CFA/LPS induced murine model. Respiratory Research.
2022;
23
(1)
:
289
.
View Article PubMed Google Scholar -
Tynecka
M.,
Janucik
A.,
Tarasik
A.,
Zbikowski
A.,
Niemira
M.,
Kulczynska-Przybik
A.,
Mesenchymal stromal cells effectively limit house dust mite extract-induced mixed granulocytic lung inflammation. Allergy.
2024;
79
(11)
:
3157-61
.
View Article PubMed Google Scholar -
Whitehead
G.S.,
Thomas
S.Y.,
Shalaby
K.H.,
Nakano
K.,
Moran
T.P.,
Ward
J.M.,
TNF is required for TLR ligand-mediated but not protease-mediated allergic airway inflammation. The Journal of Clinical Investigation.
2017;
127
(9)
:
3313-26
.
View Article PubMed Google Scholar -
Liu
J.,
Chang
G.,
Huang
J.,
Wang
Y.,
Ma
N.,
Roy
A.C.,
Sodium Butyrate Inhibits the Inflammation of Lipopolysaccharide-Induced Acute Lung Injury in Mice by Regulating the Toll-Like Receptor 4/Nuclear Factor κB Signaling Pathway. Journal of Agricultural and Food Chemistry.
2019;
67
(6)
:
1674-82
.
View Article PubMed Google Scholar -
Liu
S.,
Chen
X.,
Zhang
S.,
Wang
X.,
Du
X.,
Chen
J.,
miR‑106b‑5p targeting SIX1 inhibits TGF‑β1‑induced pulmonary fibrosis and epithelial‑mesenchymal transition in asthma through regulation of E2F1. International Journal of Molecular Medicine.
2021;
47
(3)
:
24
.
View Article PubMed Google Scholar -
Wheeler
M.J.,
Green
D.J.,
Ellis
K.A.,
Cerin
E.,
Heinonen
I.,
Naylor
L.H.,
Distinct effects of different adjuvants in the mouse model of allergic airway inflammation. Asian Pacific Journal of Allergy and Immunology.
2020;
40
(2)
:
111-120
.
View Article Google Scholar -
Heldner
A.,
Alessandrini
F.,
Russkamp
D.,
Heine
S.,
Schnautz
B.,
Chaker
A.,
Immunological effects of adjuvanted low-dose allergoid allergen-specific immunotherapy in experimental murine house dust mite allergy. Allergy.
2022;
77
(3)
:
907-19
.
View Article PubMed Google Scholar -
Shilovskiy
I.P.,
Sundukova
M.S.,
А.А. Babakhin,
Gaisina
A.R.,
Maerle
A.V.,
Sergeev
I.V.,
Experimental protocol for development of adjuvant-free murine chronic model of allergic asthma. Journal of Immunological Methods.
2019;
468
:
10-9
.
View Article PubMed Google Scholar -
Blanco
O.,
Ramírez
W.,
Lugones
Y.,
Díaz
E.,
Morejón
A.,
Rodríguez
V.S.,
Protective effects of Surfacen in allergen-induced asthma mice model. International Immunopharmacology.
2022;
102
:
108391
.
View Article PubMed Google Scholar -
Yu
Q.L.,
Chen
Z.,
Establishment of different experimental asthma models in mice. Experimental and Therapeutic Medicine.
2018;
15
(3)
:
2492-8
.
View Article PubMed Google Scholar -
Ding
F.,
Fu
Z.,
Liu
B.,
Lipopolysaccharide Exposure Alleviates Asthma in Mice by Regulating Th1/Th2 and Treg/Th17 Balance. Medical Science Monitor.
2018;
24
:
3220-9
.
View Article PubMed Google Scholar -
Liu
Y.,
Chen
Z.,
Xu
K.,
Wang
Z.,
Wu
C.,
Sun
Z.,
Next generation sequencing for miRNA profile of spleen CD4+ T cells in the murine model of acute asthma. Epigenomics.
2018;
10
(8)
:
1071-83
.
View Article PubMed Google Scholar -
Campa
C.C.,
Silva
R.L.,
Margaria
J.P.,
Pirali
T.,
Mattos
M.S.,
Kraemer
L.R.,
Inhalation of the prodrug PI3K inhibitor CL27c improves lung function in asthma and fibrosis. Nature Communications.
2018;
9
(1)
:
5232
.
View Article PubMed Google Scholar -
Gong
S.,
Ji
X.,
Su
J.,
Wang
Y.,
Yan
X.,
Wang
G.,
Yeast Fermentate Prebiotic Ameliorates Allergic Asthma, Associating with Inhibiting Inflammation and Reducing Oxidative Stress Level through Suppressing Autophagy. Mediators of Inflammation.
2021;
2021
:
4080935
.
View Article PubMed Google Scholar -
Lee
J.E.,
Im
D.S.,
Suppressive Effect of Carnosol on Ovalbumin-Induced Allergic Asthma. Biomolecules & Therapeutics.
2021;
29
(1)
:
58-63
.
View Article PubMed Google Scholar -
Huang
C.,
Zhang
Z.,
Wang
L.,
Liu
J.,
Gong
X.,
Zhang
C.,
ML-7 attenuates airway inflammation and remodeling via inhibiting the secretion of Th2 cytokines in mice model of asthma. Molecular Medicine Reports.
2018;
17
(5)
:
6293-300
.
View Article PubMed Google Scholar -
Kondo
M.,
Tezuka
T.,
Ogawa
H.,
Koyama
K.,
Bando
H.,
Azuma
M.,
Lysophosphatidic Acid Regulates the Differentiation of Th2 Cells and Its Antagonist Suppresses Allergic Airway Inflammation. International Archives of Allergy and Immunology.
2021;
182
(1)
:
1-13
.
View Article PubMed Google Scholar -
Lu
D.,
Lu
J.,
Ji
X.,
Ji
Y.,
Zhang
Z.,
Peng
H.,
IL 27 suppresses airway inflammation, hyperresponsiveness and remodeling via the STAT1 and STAT3 pathways in mice with allergic asthma. International Journal of Molecular Medicine.
2020;
46
(2)
:
641-52
.
View Article PubMed Google Scholar -
Khumalo
J.,
Kirstein
F.,
Hadebe
S.,
Brombacher
F.,
IL-4Rα signaling in CD4+CD25+FoxP3+ T regulatory cells restrains airway inflammation via limiting local tissue IL-33. JCI Insight.
2020;
5
(20)
:
e136206
.
View Article PubMed Google Scholar -
Mattos
M.S.,
Ferrero
M.R.,
Kraemer
L.,
Lopes
G.A.,
Reis
D.C.,
Cassali
G.D.,
CXCR1 and CXCR2 Inhibition by Ladarixin Improves Neutrophil-Dependent Airway Inflammation in Mice. Frontiers in Immunology.
2020;
11
:
566953
.
View Article PubMed Google Scholar -
Safar
H.A.,
El-Hashim
A.Z.,
Amoudy
H.,
Mustafa
A.S.,
Mycobacterium tuberculosis-Specific Antigen Rv3619c Effectively Alleviates Allergic Asthma in Mice. Frontiers in Pharmacology.
2020;
11
:
532199
.
View Article PubMed Google Scholar -
Camargo
L.D.,
Dos Santos
T.M.,
de Andrade
F.C.,
Fukuzaki
S.,
Dos Santos Lopes
F.D.,
de Arruda Martins
M.,
Bronchial Vascular Remodeling Is Attenuated by Anti-IL-17 in Asthmatic Responses Exacerbated by LPS. Frontiers in Pharmacology.
2020;
11
:
1269
.
View Article PubMed Google Scholar -
Zhu
F.F.,
Wang
Y.M.,
He
G.Z.,
Chen
Y.F.,
Gao
Y.D.,
Different effects of acetyl-CoA carboxylase inhibitor TOFA on airway inflammation and airway resistance in a mice model of asthma. Pharmacological Reports.
2020;
72
(4)
:
1011-20
.
View Article PubMed Google Scholar -
Sun
X.,
Hou
T.,
Cheung
E.,
Iu
T.N.,
Tam
V.W.,
Chu
I.M.,
Anti-inflammatory mechanisms of the novel cytokine interleukin-38 in allergic asthma. Cellular & Molecular Immunology.
2020;
17
(6)
:
631-46
.
View Article PubMed Google Scholar -
Wang
C.,
Liu
Q.,
Chen
F.,
Xu
W.,
Zhang
C.,
Xiao
W.,
IL-25 Promotes Th2 Immunity Responses in Asthmatic Mice via Nuocytes Activation. PLoS One.
2016;
11
(9)
:
e0162393
.
View Article PubMed Google Scholar -
Kwak
D.W.,
Park
D.,
Kim
J.H.,
Leukotriene B4 receptors play critical roles in house dust mites-induced neutrophilic airway inflammation and IL-17 production. Biochemical and Biophysical Research Communications.
2021;
534
:
646-52
.
View Article PubMed Google Scholar -
Sun
Z.,
Ji
N.,
Ma
Q.,
Zhu
R.,
Chen
Z.,
Wang
Z.,
Epithelial-Mesenchymal Transition in Asthma Airway Remodeling Is Regulated by the IL-33/CD146 Axis. Frontiers in Immunology.
2020;
11
:
1598
.
View Article PubMed Google Scholar -
Khumalo
J.,
Kirstein
F.,
Scibiorek
M.,
Hadebe
S.,
Brombacher
F.,
Therapeutic and prophylactic deletion of IL-4Ra-signaling ameliorates established ovalbumin induced allergic asthma. Allergy.
2020;
75
(6)
:
1347-60
.
View Article PubMed Google Scholar -
Raemdonck
K.,
Baker
K.,
Dale
N.,
Dubuis
E.,
Shala
F.,
Belvisi
M.G.,
CD4+ and CD8+ T cells play a central role in a HDM driven model of allergic asthma. Respiratory Research.
2016;
17
(1)
:
45
.
View Article PubMed Google Scholar -
Luo
W.,
Hu
J.,
Xu
W.,
Dong
J.,
Distinct spatial and temporal roles for Th1, Th2, and Th17 cells in asthma. Frontiers in Immunology.
2022;
13
:
974066
.
View Article PubMed Google Scholar -
Ahuja
S.K.,
Manoharan
M.S.,
Harper
N.L.,
Jimenez
F.,
Hobson
B.D.,
Martinez
H.,
Preservation of epithelial cell barrier function and muted inflammation in resistance to allergic rhinoconjunctivitis from house dust mite challenge. The Journal of Allergy and Clinical Immunology.
2017;
139
(3)
:
844-54
.
View Article PubMed Google Scholar -
Drake
M.G.,
Scott
G.D.,
Blum
E.D.,
Lebold
K.M.,
Nie
Z.,
Lee
J.J.,
Eosinophils increase airway sensory nerve density in mice and in human asthma. Science Translational Medicine.
2018;
10
(457)
:
eaar8477
.
View Article PubMed Google Scholar -
Koopmans
T.,
Hesse
L.,
Nawijn
M.C.,
Kumawat
K.,
Menzen
M.H.,
Sophie T Bos
I.,
Smooth-muscle-derived WNT5A augments allergen-induced airway remodelling and Th2 type inflammation. Scientific Reports.
2020;
10
(1)
:
6754
.
View Article PubMed Google Scholar -
Pan
J.,
Yang
Q.,
Zhou
Y.,
Deng
H.,
Zhu
Y.,
Zhao
D.,
MicroRNA-221 Modulates Airway Remodeling via the PI3K/AKT Pathway in OVA-Induced Chronic Murine Asthma. Frontiers in Cell and Developmental Biology.
2020;
8
:
495
.
View Article PubMed Google Scholar -
Dong
L.,
Wang
Y.,
Zheng
T.,
Pu
Y.,
Ma
Y.,
Qi
X.,
Hypoxic hUCMSC-derived extracellular vesicles attenuate allergic airway inflammation and airway remodeling in chronic asthma mice. Stem Cell Research & Therapy.
2021;
12
(1)
:
4
.
View Article PubMed Google Scholar -
Mazarakis
N.,
Vongsvivut
J.,
Bambery
K.R.,
Ververis
K.,
Tobin
M.J.,
Royce
S.G.,
Investigation of molecular mechanisms of experimental compounds in murine models of chronic allergic airways disease using synchrotron Fourier-transform infrared microspectroscopy. Scientific Reports.
2020;
10
(1)
:
11713
.
View Article PubMed Google Scholar -
Akkoc
T.,
O'Mahony
L.,
Ferstl
R.,
Akdis
C.,
Akkoc
T.,
Mouse Models of Asthma: Characteristics, Limitations and Future Perspectives on Clinical Translation. In: Turksen, K. (eds) Cell Biology and Translational Medicine, Volume 15. Advances in Experimental Medicine and Biology(), vol 1376. Springer, Cham. 2021;
:
119-33
.
View Article Google Scholar -
Rosshart
S.P.,
Herz
J.,
Vassallo
B.G.,
Hunter
A.,
Wall
M.K.,
Badger
J.H.,
Laboratory mice born to wild mice have natural microbiota and model human immune responses. Science.
2019;
365
(6452)
:
365
.
View Article PubMed Google Scholar -
Alessandrini
F.,
Musiol
S.,
Schneider
E.,
Blanco-Pérez
F.,
Albrecht
M.,
Mimicking Antigen-Driven Asthma in Rodent Models-How Close Can We Get?. Frontiers in Immunology.
2020;
11
:
575936
.
View Article PubMed Google Scholar -
Chen
C.C.,
Kobayashi
T.,
Iijima
K.,
Hsu
F.C.,
Kita
H.,
IL-33 dysregulates regulatory T cells and impairs established immunologic tolerance in the lungs. The Journal of Allergy and Clinical Immunology.
2017;
140
(5)
:
1351-1363.e7
.
View Article PubMed Google Scholar -
Kobayashi
T.,
Iijima
K.,
Matsumoto
K.,
Lama
J.K.,
Kita
H.,
Lung-resident CD69+ST2+ TH2 cells mediate long-term type 2 memory to inhaled antigen in mice. The Journal of Allergy and Clinical Immunology.
2023;
152
(1)
:
167-181.e6
.
View Article PubMed Google Scholar -
Chen
J.C.,
Chan
C.C.,
Wu
C.J.,
Ou
L.S.,
Yu
H.Y.,
Chang
H.L.,
Fetal Phagocytes Take up Allergens to Initiate T-Helper Cell Type 2 Immunity and Facilitate Allergic Airway Responses. American Journal of Respiratory and Critical Care Medicine.
2016;
194
(8)
:
934-47
.
View Article PubMed Google Scholar -
Bracken
S.J.,
Adami
A.J.,
Rafti
E.,
Schramm
C.M.,
Matson
A.P.,
Regulation of IgE activity in inhalational tolerance via formation of IgG anti-IgE/IgE immune complexes. Clinical and Molecular Allergy : CMA.
2018;
16
(1)
:
13
.
View Article PubMed Google Scholar -
Wang
J.,
Zhou
Y.,
Zhang
H.,
Hu
L.,
Liu
J.,
Wang
L.,
Pathogenesis of allergic diseases and implications for therapeutic interventions. Signal Transduction and Targeted Therapy.
2023;
8
(1)
:
138
.
View Article PubMed Google Scholar -
Li
H.,
Bi
Q.,
Cui
H.,
Lv
C.,
Wang
M.,
Suppression of autophagy through JAK2/STAT3 contributes to the therapeutic action of rhynchophylline on asthma. BMC Complementary Medicine and Therapies.
2021;
21
(1)
:
21
.
View Article PubMed Google Scholar -
Wu
C.T.,
Lin
F.H.,
Lee
Y.T.,
Ku
M.S.,
Lue
K.H.,
Effect of Lactobacillus rhamnosus GG immunopathologic changes in chronic mouse asthma model. Journal of Microbiology, Immunology, and Infection = Wei Mian Yu Gan Ran Za Zhi.
2019;
52
(6)
:
911-9
.
View Article PubMed Google Scholar -
Vroman
H.,
Bergen
I.M.,
Hulst
J.A. van,
Nimwegen
M. van,
Uden
D. van,
Schuijs
M.J.,
TNF-α-induced protein 3 levels in lung dendritic cells instruct TH2 or TH17 cell differentiation in eosinophilic or neutrophilic asthma. The Journal of Allergy and Clinical Immunology.
2018;
141
(5)
:
1620-1633.e12
.
View Article PubMed Google Scholar -
Li
S.,
Miao
Z.,
Tian
Y.,
Wang
H.,
Wang
S.,
He
T.,
Limethason reduces airway inflammation in a murine model of ovalbumin-induced chronic asthma without causing side effects. Experimental and Therapeutic Medicine.
2018;
15
(3)
:
2269-76
.
View Article PubMed Google Scholar -
Turner
D.L.,
Goldklang
M.,
Cvetkovski
F.,
Paik
D.,
Trischler
J.,
Barahona
J.,
Biased Generation and In Situ Activation of Lung Tissue-Resident Memory CD4 T Cells in the Pathogenesis of Allergic Asthma. The Journal of Immunology : Official Journal of the American Association of Immunologists.
2018;
200
(5)
:
1561-9
.
View Article PubMed Google Scholar -
Choi
J.Y.,
Lee
H.Y.,
Hur
J.,
Kim
K.H.,
Kang
J.Y.,
Rhee
C.K.,
TRPV1 Blocking Alleviates Airway Inflammation and Remodeling in a Chronic Asthma Murine Model. Allergy, Asthma & Immunology Research.
2018;
10
(3)
:
216-24
.
View Article PubMed Google Scholar -
Lee
Y.T.,
Wu
C.T.,
Sun
H.L.,
Ko
J.L.,
Lue
K.H.,
Fungal immunomodulatory protein-fve could modulate airway remodel through by affect IL17 cytokine. Journal of Microbiology, Immunology, and Infection = Wei Mian Yu Gan Ran Za Zhi.
2018;
51
(5)
:
598-607
.
View Article PubMed Google Scholar -
Turner
P.V.,
Pang
D.S.,
Lofgren
J.L.,
A Review of Pain Assessment Methods in Laboratory Rodents. Comparative Medicine.
2019;
69
(6)
:
451-67
.
View Article PubMed Google Scholar
Comments
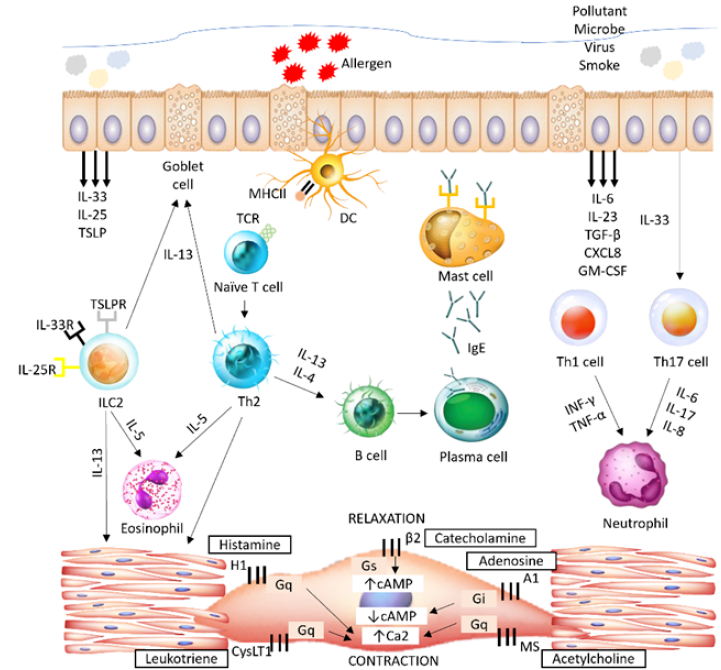
Article Details
Volume & Issue : Vol 12 No 4 (2025)
Page No.: 7320-7334
Published on: 2025-04-30
Citations
Copyrights & License

This work is licensed under a Creative Commons Attribution 4.0 International License.
Search Panel
- HTML viewed - 448 times
- PDF downloaded - 130 times
- XML downloaded - 34 times