Abstract
Introduction: Stem cell-based therapies for Parkinson's disease (PD) represent a promising frontier in regenerative medicine. This study assesses the efficacy of regenerative treatments, specifically platelet-rich plasma (PRP) and peripheral blood-derived very small embryonic-like (PBD-VSEL) stem cell therapy, in managing PD.
Methods: A quasi-experimental study design was employed, involving 50 PD patients divided into two cohorts. Group A (n = 25) received only standard therapy, while Group B (n = 25) received the addition of PRP and PBD-VSEL stem cell therapy to the standard treatment. The primary outcomes measured were changes in the Unified Parkinson's Disease Rating Scale (UPDRS) and the Parkinson's Disease Questionnaire-39 (PDQ-39) scores.
Results: The average age was 61.40 years in Group A and 62.16 years in Group B. At baseline, both groups exhibited comparable UPDRS and PDQ-39 scores. However, at the 6-month follow-up, Group B demonstrated greater improvement in PDQ-39 scores (64.16 ± 5.44) compared to Group A (71.64 ± 10.68). After one year, Group B presented significantly reduced UPDRS (60.80 ± 5.85) and PDQ-39 (59.88 ± 5.12) scores relative to Group A (69.44 ± 9.67 and 66.52 ± 5.52), highlighting superior enhancements in motor function and quality of life for Group B.
Conclusion: The findings suggest that PRP and PBD-VSEL stem cells from peripheral blood may offer a viable adjunctive treatment for Parkinson's disease. Nonetheless, additional research is essential to elucidate the mechanisms underlying cell therapy and substantiate its clinical application. Cell transplantation appears to be a safer and more efficacious strategy for treating Parkinson's disease. The management of advanced PD patients requires careful decision-making, including the introduction of new pharmacotherapies and adjustments to levodopa dosages. Larger studies with extended follow-up periods are necessary to confirm these results.
Introduction
Parkinson's disease (PD) is a neurodegenerative disorder characterized by the degeneration of mesencephalic dopaminergic neurons within the substantia nigra, leading to symptoms such as bradykinesia, resting tremor, and rigidity predominantly affecting the body's central nervous system (CNS) related to motor control1. Over the past 25 years, the prevalence of PD has increased twofold. On a global scale, PD-related disability and mortality rates are rising more rapidly compared to other neurological conditions1, 2. PD can be classified into juvenile, early, or late-onset categories. Juvenile cases are rare, usually familial, and often associated with mutations in the Parkin gene3. Generally, PD impacts approximately 1% of the population over the age of 60 and 4% of individuals over the age of 804. The clinical manifestations of PD are a consequence of dopamine deficiency and the progressive degeneration of dopaminergic neurons (DAn)5, 6. The neurodegenerative effects of PD may extend beyond motor functions, affecting both motor and non-motor regions of the body7. The primary pathological feature of this neurodegeneration is the loss of dopaminergic neurons in the substantia nigra pars compacta, resulting in reduced dopamine levels in the neostriatum8, 9.
L-DOPA (L-3,4-dihydroxyphenylalanine), a precursor to dopamine, serves as the primary therapeutic agent for PD, providing immediate symptomatic relief. However, it often leads to motor fluctuations after 5-10 years of use 10. Although levodopa remains the gold standard treatment for symptom relief, developing neuroprotective strategies is essential for creating therapies that more specifically target the underlying pathology11. As neurodegeneration advances, significant alterations occur in both brain and body, with organs, tissues, and cells communicating these changes via systemic signaling pathways12. Despite L-DOPA administration, PD patients may experience refractory dyskinesia. Newer treatments, such as dopamine agonists and deep brain stimulation, help address these challenges but do not prevent disease progression or alleviate symptoms resulting from non-dopaminergic pathologies13.
Numerous clinical trials have demonstrated that plasma administration can enhance cognitive function in aging rodents14, 15, 16. This suggests that circulating plasma components can ameliorate cognitive and disease-related symptoms17. This has encouraged the exploration of blood products and their active components for treating various disorders, particularly those related to dementia or neurodegeneration. The limitations of pharmaceutical interventions have spurred interest in developing alternative treatments18. Human embryonic dopaminergic neurons have been investigated in clinical trials for cell replacement therapy with some success19, 20. However, promising clinical results and evidence of graft survival remain limited. Intracerebral xenografts require strict immunosuppression and pose a risk of transmitting animal viruses21. The loss of midbrain neurons implies that regenerating a single brain region with new cells may mitigate these deficits, positioning PD as a pioneer in cell-based therapy22. Due to the non-targeted and non-physiological distribution of dopamine in the brain, current therapies, although alleviating pyramidal symptoms, have significant side effects18, 23. As dopamine deficiency underlies PD symptoms, cell transplantation offers potential symptomatic relief without the pyramidal and neuropsychiatric side effects associated with dopaminergic drugs24. Over the last twenty years, regenerative cell therapy has opened unprecedented avenues for innovative strategies to combat neurodegenerative diseases25.
Human stem cells, specifically multipotent region-specific and pluripotent embryonic stem cells, are considered potential sources of dopaminergic neurons for PD treatment through cell transplantation26. These transplanted neurons must meet certain criteria, including proper synthesis and release of dopamine, reinnervation of the striatum, and mitigation of motor symptoms27. Very small embryonic-like cells (VSELs), identified as putative pluripotent stem cells in human umbilical cord blood (HUCB) and mouse bone marrow, hold significant promise for regenerative medicine applications28, 29. Recent studies suggest that effective dopaminergic neurons should express the G-protein-coupled inward rectifying K+ channel subunit (Girk2), predominantly found in the substantia nigra pars compacta30. Stem cell-based therapies offer a promising pathway for developing effective regenerative medicine benefiting a broad population14, 31. Despite limited research on the use of PRP and PBD-VSEL stem cells for PD treatment, stem cells are likely to find clinical application soon. While progress remains gradual, several promising clinical trials are underway, indicating that stem cell therapy may soon be viable for PD management. The current study aims to assess the efficacy of PRP and stem cell therapies in managing PD.
Methods
Patient Enrollment with Inclusion and Exclusion Criteria
This quasi-experimental study received approval from the Research Ethics Committee at The University of Lahore, Pakistan (IRB-SPRM-2020-2), with input from a review committee comprised of expert neurologists and neuropathologists from the University of Jeddah and King Abdulaziz University in Jeddah, Saudi Arabia. Informed written consent was obtained from either the patient or the patient's legal guardian if the patient was unable to communicate. The study is registered at ClinicalTrials.gov (NCT06142981), which can be accessed at https://clinicaltrials.gov/study/NCT06142981. All participants, clinically diagnosed with Parkinson's Disease (PD), were referred from the Institute of Neurosciences, Lahore, Pakistan (Figure 1). The sample size calculation, using a 21.7% prevalence rate of PD, a margin of error of 8%, an 80% confidence level, and a 10% dropout rate, resulted in an estimated sample size of 50 participants32. Patients were comprehensively briefed about the treatment procedures, inclusive of potential risks, benefits, complications, and long-term effects. Data on each patient's medical history, PD stage, symptom characteristics, previous treatments, and comorbidities were collected through interviews and retrospective chart reviews. Following treatment, participants were monitored over two years. Inclusion criteria focused on individuals with a PD diagnosis spanning 4 to 10 years, aged 50 to 70, of either gender, and on stable treatment regimens. Exclusion criteria ruled out individuals with gout, congestive heart failure, renal failure, uncontrolled atrial fibrillation, stroke, anaphylaxis, coagulopathies, and those with clinical suspicion or confirmed diagnosis of atypical Parkinsonism or essential tremor.
Clinical Assessment of PD Treatment Response
Neurologists conducted clinical assessments using recognized scales to evaluate the response to PD treatment. The primary tool utilized was the Unified Parkinson's Disease Rating Scale (UPDRS), a prominent rating scale from the 1980s for tracking PD progression2. Additionally, the self-report Parkinson's Disease Questionnaire–39 (PDQ-39) was employed, which is a specific health status questionnaire for PD encompassing 39 items, asking participants to rate the frequency of experienced difficulties related to their PD on a five-point scale33.
Treatment Method
The study enrolled 50 patients, split into two equal groups. Group A (n = 25) received standard Parkinson's disease therapy, while Group B (n = 25) was treated with Standard Therapy plus Regenerative Medicine (PRP and PBD-VSEL Stem Cell Therapy). Post initial clinical assessment by neurologists, patients were enrolled in a treatment plan and, following cognitive and laboratory testing, scheduled for treatment infusion within two weeks (Figure 1). Group A received a daily dose of 300 mg levodopa, administered over three doses and adjusted based on individual response, alongside lifestyle modification therapy. Group B received PRP injections at four acupuncture points (ST36 and GB 34, bilaterally) 34, 35, 36, followed by PBD-VSEL stem cell administration at these points post-PRP sessions, with some patients receiving intra-discal injections for targeted spinal intervention to enhance regenerative outcomes. Following PRP sessions, patients were re-evaluated by neurologists, with PBD-VSEL stem cell therapy administered at the 90-day mark. Evaluations continued at 3-month intervals, with PRP booster doses provided at 6 and 9 months post-cell therapy, followed by annual evaluations and monitoring for side effects.
Single Syringe Method for PRP Isolation from Blood
Venous blood drawn was centrifuged at 2800 rpm for 10 minutes using a soft spin, dividing into 60 mL and 140 mL portions. From the 60 mL portion, 10–12 mL PRP was extracted and combined with the remaining 140 mL autologous blood. During sessions, 4 mL of PRP mix was injected at four acupuncture sites.
Peripheral Blood-Derived Very Small Embryonic-Like (PBD-VSEL) Stem Cells Preparation and Regimen
120 mL of peripheral venous blood was drawn for processing. 30 mL was used for VSEL isolation, with remaining blood discarded after plasma separation or reserved for further analysis. VSELs isolated via centrifugation at 600×g and 1200×g steps, were purified, exposed to monochromatic light to boost regenerative potential before re-administration. Post-isolation, sterility tests were done to ensure the absence of contamination before injection, with evaluations on cell viability using a Muse Cell Analyzer (Merck, Millipore, USA). Internal tests showed stable cell count and viability for 24 hours at 2-8°C. The corrected mention aligns with study goals. Injection procedures adhered to sterile standards with live C-arm fluoroscopic guidance and consistent physician oversight. Post-procedural follow-up assessed infection risk and pain management efficacy37, 38.
Primary Outcome and Statistical Analysis
Follow-ups for both groups were conducted at baseline, the 6th month, and 1-year intervals. Primary outcomes measured improvements via UPDRS, as the main scale for PD progression, and PDQ-39 health questionnaire responses. Data analysis was done using SPSS 25.0 with differences assessed via independent sample t-tests, with significance set at p ≤ 0.05. ANOVA analysis contributed to statistical validation.
Results
The study involved 50 patients, equally divided into Group A (n = 25) and Group B (n = 25). The mean age was 61.40 years in Group A and 62.16 years in Group B. The average duration of Parkinson's disease was comparable between the groups, with 7.32 years in Group A and 6.76 years in Group B, indicating that both groups had experienced the disease for similar periods. Gender distribution was also similar, with Group A comprising 60% males and 40% females, whereas Group B included 64% males and 36% females. A notable difference was observed in family history, with 28% of Group A reporting a family history of Parkinson's disease compared to 60% in Group B. In terms of motor subtype dominance, tremor-dominant patients were more prevalent, especially in Group B (80%) as opposed to Group A (64%). In contrast, Group A had a higher proportion of patients with postural instability and gait difficulty (36%) compared to Group B (20%) (Table 1).
Group A | Group B | |
Age (Mean +SD) | 61.40+4.70 | 62.16+6.61 |
Duration of disease (years) | 7.32+1.99 | 6.76+1.66 |
Gender | f (%) | f (%) |
Male | 15(60.0) | 16(64.0) |
Female | 10(40.0) | 9(36.0) |
Family history | ||
Yes | 7(28.0) | 15(60.0) |
No | 18(72.0) | 10(40.0) |
Motor Subtype dominant | ||
Postural instability and gait difficulty (PIGD) | 9(36.0) | 5(20.0) |
tremor-dominant (TD) | 16(64.0) | 20(80.0) |
Specific Area of Motor Symptoms | ||
Head | 7(28.0) | 8(32.0) |
Arm | 14(56.0) | 13(52.0) |
Leg | 4(16.0) | 4(16.0) |
Variable | Groups | Mean+ SD | P-value |
UPDRS at baseline | Group A | 99.52+13.60 | 0.469 |
Group B | 96.36+16.86 | ||
PDQ-39 at baseline | Group A | 74.24+8.55 | 0.491 |
Group B | 76.0+9.35 | ||
UPDRS at 6 th Month | Group A | 88.60+13.46 | 0.165 |
Group B | 82.92+15.0 | ||
PDQ-39 at 6 th Month | Group A | 71.64+10.68 | 0.003* |
Group B | 64.16+5.44 | ||
UPDRS at 1 Year | Group A | 69.44+9.67 | 0.000* |
Group B | 60.80+5.85 | ||
PDQ-39 at 1 Year | Group A | 66.52+5.52 | 0.000* |
Group B | 59.88+5.12 |
Effectiveness of treatment
The comparison of outcomes between Group A and Group B revealed notable differences over time. Initially, the UPDRS scores (Unified Parkinson's Disease Rating Scale) were similar, with Group A averaging 99.52 ± 13.60 and Group B 96.36 ± 16.86, a difference that was not statistically significant (p = 0.469). The baseline PDQ-39 scores (Parkinson’s Disease Questionnaire) were also similar, with Group A at 74.24 ± 8.55 and Group B at 76.0 ± 9.35, with no significant difference (p = 0.491).
At the 6-month follow-up, UPDRS scores showed improvement in both groups, with Group A at 88.60 ± 13.46 and Group B at 82.92 ± 15.0, although the difference remained statistically insignificant (p = 0.165). There was, however, a significant improvement in PDQ-39 scores at 6 months, with Group B showing a greater reduction (64.16 ± 5.44) than Group A (71.64 ± 10.68), resulting in a statistically significant difference (p = 0.003). By the 1-year follow-up, the differences between the groups became more prominent. Group B had significantly lower UPDRS scores (60.80 ± 5.85) compared to Group A (69.44 ± 9.67), with the difference achieving statistical significance (p = 0.000). Similarly, PDQ-39 scores also improved more in Group B (59.88 ± 5.12) than in Group A (66.52 ± 5.52), with a significant difference (p = 0.000). These findings suggest that Group B showed greater improvements in both motor function and quality of life over time compared to Group A (Table 2). A significant main effect of time was observed, F(1.694, 81.292) = 80.683, p < 0.001, η² = 0.627, indicating overall improvement in motor function. However, the time × group interaction was not significant, F(1.694, 81.292) = 0.551, p = 0.550, η² = 0.011, suggesting similar changes over time in both groups. Pairwise comparisons with Bonferroni adjustment showed significant UPDRS score improvements between baseline and 1 year (p < 0.001), with no significant differences at 6 months (p = 0.115). The repeated measures ANOVA for PDQ-39 scores showed a significant main effect of time (F(1.954, 93.798) = 30.810, p < 0.001, η² = 0.391), indicating substantial improvement in quality of life over time. Additionally, a significant time × group interaction (F(1.954, 93.798) = 11.740, p < 0.001, η² = 0.197) suggests variation in improvement extent between treatment groups, with Group B experiencing greater benefits in quality of life over time (Table 3, Table 4 ).
Effect | SS | df | MS | F | p-value | Partial η² |
---|---|---|---|---|---|---|
Time | 27,525.240 | 1.694 | 16,252.686 | 80.683 | <0.001 | 0.627 |
Time × Group | 188.093 | 1.694 | 111.062 | 0.551 | 0.550 | 0.011 |
Error (Time) | 16,375.333 | 81.292 | 201.439 | — | — | — |
Gender-based comparison and follow-up
The gender-based comparison of UPDRS and PDQ-39 scores at baseline, 6 months, and 1 year showed no statistically significant differences between males and females (p > 0.05). At baseline, males had a mean UPDRS score of 94.74, while females had a higher score of 103.16. For PDQ-39, males scored 73.71, and females scored 77.42. By the 6-month mark, UPDRS scores improved for both genders, with males scoring 85.52 and females 86.16, while PDQ-39 scores were nearly identical, at 69.90 for males and 69.43 for females. By the 1-year follow-up, UPDRS scores further improved, with males at 65.06 and females at 65.21. PDQ-39 scores at 1 year also showed minimal difference, with males at 63.19 and females at 63.21. Overall, both genders showed improvements in motor function and quality of life, but the absence of significant differences indicates that gender did not substantially affect these outcomes (Figure 2).
Effect | SS | df | MS | F | p-value | Partial η² |
---|---|---|---|---|---|---|
Time | 4,164.520 | 1.954 | 2,131.146 | 30.810 | <0.001 | 0.391 |
Time × Group | 1,586.813 | 1.954 | 812.034 | 11.740 | <0.001 | 0.197 |
Error (Time) | 6,488.000 | 93.798 | 69.170 | — | — | — |
Discussion
Over a two-year period, most Parkinson's Disease (PD) patients remained stable, exhibiting mild to moderate symptoms and maintaining physical independence according to specific tests and patient surveys. The Parkinson's Disease Questionnaire-39 (PDQ-39) results demonstrated a moderate impact of PD on functioning and well-being, aligning with previous studies33. Management of PD primarily involves medication, with L-dopa being the most frequently prescribed drug available in various forms. Another treatment option is deep brain stimulation (DBS) surgery, although it is not regarded as the optimal solution39. It is crucial to recognize that these treatments solely manage symptoms without addressing the disease's underlying biology, offering a long-term solution, or halting the progression of degeneration40. Stem cells represent a promising avenue for dopamine (DA) regeneration, prompting ongoing research efforts aiming to develop more effective treatments for PD41. Platelet-rich plasma (PRP), as a novel therapeutic hypothesis for central nervous system disorders, offers several advantages. Derived from autologous blood, PRP minimizes infection and immune rejection risks. The formulation's simplicity and cost-effectiveness facilitate clinical application. Additionally, platelet-rich growth factors (PRGFs) enhance neurotrophic effects by promoting natural recombination with other growth factors. As an indigenous tissue engineering scaffold, PRG also ensures biocompatibility42.
Our research revealed that PRP and peripheral blood-derived viable stem cell-enriched leukocytes (PBD-VSEL) stem cell therapy resulted in significant improvements in depressive symptoms, quality of life, and symptom relief. Baseline Unified Parkinson's Disease Rating Scale (UPDRS) scores averaged 97.3 ± 14.9, which significantly decreased at both 1-year and 2-year follow-ups (72.9 ± 9.7 and 64.5 ± 10.0, respectively), indicating notable patient condition improvement after treatment. These outcomes were comparable to a prospective pilot study assessing the effects of a single-dose unilateral transplantation of autologous bone marrow-derived mesenchymal stem cells (BM-MSCs) in PD patients, which reported patient follow-up ranging from 10 to 36 months with an average baseline UPDRS score of 65 ± 22.06. Findings highlighted that 3 out of 7 patients consistently improved their UPDRS scores over time, reflecting a total improvement of 22.9% from baseline43. In another study, embryonic dopamine neurons were employed in PD treatment, with patients randomly assigned to either receive nerve cell transplants or undergo a placebo operation. At the one-year follow-up, mean (SD) improvement or worsening ratings on the overall scale were 0.02 ± 1.1 in the transplant group and -0.41 ± 1.7 in the placebo group. Younger transplant recipients notably experienced greater improvement in UPDRS ratings compared to the placebo group (P = 0.01)24. Current findings were consistent with a meta-analysis regarding cellular treatment's impact on motor symptoms in PD patients before and after cell transplantation, indicating symptomatic relief44.
Current study results indicate a baseline PDQ-39 score of 75.1 ± 8.62, which progressively improved at 1 and 2 years post-treatment (43.9 ± 9.85 and 30.3 ± 8.91, respectively) with statistically significant enhancement (p < 0.05). Additionally, patients reported significant improvement in the Hospital Anxiety and Depression Scale (HADS), with a substantial decrease in anxiety post-treatment (p < 0.05). Comparative analysis with another study evaluating autologous mesenchymal stem cell (MSC) therapy's effectiveness in ameliorating both motor and non-motor symptoms of PD showed a 9% reduction in UPDRS scores post-treatment. Additionally, the study indicated improved sleep quality, reduced daytime drowsiness, and elevated mood, with a 44% improvement in mood and decreased depressive symptoms after three months (P = 0.01). The PDQ-39 scale also reflected statistically significant quality of life enhancement (P = 0.003), with the desired outcomes persisting for three months post-transplant (P = 0.01)45.
Findings demonstrate significant enhancements in UPDRS and PDQ-39 scores for Group B at the 1-year mark, underscoring the efficacy of combining standard therapy with regenerative medicine (PRP and PBD-VSEL stem cells). However, the absence of significant UPDRS alterations at 6 months suggests delayed motor improvement, which may be attributed to the gradual neuroprotective effects of PRP and PBD-VSEL stem cells. These therapies facilitate dopaminergic neuron survival, synaptic plasticity enhancement, and axonal remodeling, processes integral to functional recovery over time46. Additionally, neuroinflammation and oxidative stress modulation by these therapies potentially contribute to sustained long-term improvements rather than immediate symptom alleviation47.
Future investigations should focus on neuronal repair biomarkers and imaging-based synaptic change assessments to further elucidate underlying mechanisms. Although stem cell therapy seeks to replenish dopaminergic neurons in the substantia nigra, differentiation and integration into functional neural circuits is a gradual process. This delay may elucidate why UPDRS improvements manifest over time. Research suggests that mesenchymal stem cells (MSCs) and other regenerative therapies activate endogenous repair mechanisms, which require prolonged periods to translate into clinical benefits.
Transplantation in PD patients showed no significant adverse effects, with patients exhibiting consistent improvements in their UPDRS scores. The treatment was deemed safe and effective, albeit the uncontrolled study design did not confirm efficacy. There is an urgent need for larger-scale trials to establish effectiveness38.
Acknowledgment of study limitations is essential. Treating advanced PD is challenging due to levodopa-associated complications, patient age, comorbidities, polypharmacy, and diverse motor and non-motor symptoms. The small sample size limits result generalizability, and the study's focus on motor symptom severity in PD may oversimplify the disease complexity. Although a reduction in UPDRS scores from 97.3 to 64.5 over two years suggests substantial motor function improvement, the study insufficiently investigates surrogate indicators, such as neuroimaging data or biomarkers, which could provide a more comprehensive assessment of disease progression and treatment efficacy.
Conclusions
Our research indicates that PRP and PBD-VSEL stem cells derived from peripheral blood can be combined for the treatment of Parkinson's Disease (PD). However, to fully understand the potential mechanisms of cell therapy and to facilitate the application of cell-based therapies in clinical settings, further research is necessary. According to the study's findings, cell transplantation represents a pioneering approach to treating PD, offering greater safety and effectiveness compared to earlier treatments. Despite the availability of various therapeutic options beyond standard dopamine replacement therapy, managing patients with advanced Parkinson's disease remains challenging. Clinicians and patients must decide when to introduce new medications into treatment regimens, adjust levodopa dosages, and select the most appropriate advanced therapies. To validate these findings, studies with larger sample sizes and extended follow-up periods are needed.
Abbreviations
BM-MSCs: Bone Marrow-Derived Mesenchymal Stem Cells; CNS: Central Nervous System; DAn: Dopaminergic Neurons; DBS: Deep Brain Stimulation; Girk2: G-protein-coupled Inward Rectifying K+ Channel Subunit; HADS: Hospital Anxiety and Depression Scale; HUCB: Human Umbilical Cord Blood; IRB: Institutional Review Board; L-DOPA: L-3,4-dihydroxyphenylalanine; MSCs: Mesenchymal Stem Cells; PBD-VSEL: Peripheral Blood-Derived Very Small Embryonic-Like; PD: Parkinson's Disease; PDQ-39: Parkinson's Disease Questionnaire-39; PRGFs: Platelet-Rich Growth Factors; PRP: Platelet-Rich Plasma; UPDRS: Unified Parkinson's Disease Rating Scale; VSEL: Very Small Embryonic-Like Cells.
Acknowledgments
None.
Author’s contributions
Shahzad Anwar: Study design, Conceptualization; Methodology; Fatima Tirmzi and Amber Hassan: Patients data, collection, methodology, investigation and analysis; Alaa Alkhotani: Pathological and Investigational interpretation; Ahmed Bamaga, Saleh Baeesa, Ahmed Najjar: Clinical Interpretation, Mohammed Karami, Adnan Badahdah, Mazen Basheikh: Editing, review, discussion and profuse writing; Humira Waseem, Gull-a-Rukh Shaukat and Amr Alkhotani; Diagram and statistical analysis; Maher Kurdi: Project supervision. All authors have critically reviewed and approved the final draft and are responsible for the content and similarity index of the manuscript.
Funding
None.
Availability of data and materials
The datasets generated for this study are available upon request from the corresponding author [MK].
Ethics approval and consent to participate
Our study was approved by the A Research Ethics Committee at The University of Lahore in Pakistan (IRB-SPRM-2020-2) and in correlation with the reviewing committee from expert neurologists and neuropathologists from the University of Jeddah and King Abdulaziz University, Jeddah, Saudi Arabia. Informed consent was obtained from all participants in the study.
Consent for publication
Not applicable.
Competing interests
The authors declare that they have no competing interests.
References
-
Bloem
B.R.,
Okun
M.S.,
Klein
C.J.,
Parkinson's disease. The Lancet.
2021;
397
(10291)
:
2284-303
.
View Article Google Scholar -
Tinelli
M.,
Kanavos
P.,
Grimaccia
F.,
The value of early diagnosis and treatment in parkinson's disease: A literature review of the potential clinical and socioeconomic impact of targeting unmet needs in Parkinson’s disease. The London School of Economics and Political Science. 2016
.
-
Kolicheski
A.,
Turcano
P.,
Tamvaka
N.,
McLean
P.J.,
Springer
W.,
Savica
R.,
Early-onset Parkinson’s disease: Creating the right environment for a genetic disorder. Journal of Parkinson’s Disease.
2022;
12
(8)
:
2353-67
.
View Article Google Scholar -
Dumurgier
J.,
C. Tzourio,
Epidemiology of neurological diseases in older adults. Revue neurologique.
2020;
176
(9)
:
642-8
.
View Article Google Scholar -
Carmichael
K.,
Sullivan
B.,
Lopez
E.,
Sun
L.,
Cai
H.J.A.,
Diverse midbrain dopaminergic neuron subtypes and implications for complex clinical symptoms of Parkinson’s disease. Ageing and neurodegenerative diseases.
2021;
1
(4)
:
10-20517
.
View Article Google Scholar -
Kumar
S.,
Goyal
L.,
Singh
S.J.C.,
Targets
N.D.-D.,
Tremor and rigidity in patients with Parkinson’s disease: emphasis on epidemiology, pathophysiology and contributing factors. CNS & Neurological Disorders-Drug Targets-CNS & Neurological Disorders.
2022;
21
(7)
:
596-609
.
View Article Google Scholar -
LeWitt
P.A.,
Chaudhuri
K.R.J.P.,
Disorders
R.,
Unmet needs in Parkinson disease: Motor and non-motor. Parkinsonism & Related Disorders.
2020;
80
:
S7-12
.
View Article Google Scholar -
Ni
A.,
C. Ernst,
Evidence that substantia nigra pars compacta dopaminergic neurons are selectively vulnerable to oxidative stress because they are highly metabolically active. Frontiers in cellular neuroscience.
2022;
16
:
826193
.
View Article Google Scholar -
Radad
K.,
Moldzio
R.,
Krewenka
C.,
Kranner
B.,
W.D. Rausch,
Pathophysiology of non-motor signs in Parkinsons disease: some recent updating with brief presentation. Exploration of Neuroprotective Therapy.
2023;
3
(1)
:
24-46
.
View Article Google Scholar -
Fabbrini
A.,
A. Guerra,
Pathophysiological mechanisms and experimental pharmacotherapy for L-dopa-induced dyskinesia. Journal of experimental pharmacology.
2021;
13
:
469-85
.
View Article Google Scholar -
Biase
L. di,
Pecoraro
P.M.,
Carbone
S.P.,
Caminiti
M.L.,
Levodopa-induced dyskinesias in Parkinson’s disease: an overview on pathophysiology, clinical manifestations, therapy management strategies and future directions. Journal of Clinical Medicine.
2023;
12
(13)
:
4427
.
View Article Google Scholar -
Braun
M.,
J.J. Iliff,
The impact of neurovascular, blood-brain barrier, and glymphatic dysfunction in neurodegenerative and metabolic diseases. International review of neurobiology.
2020;
154
:
413-36
.
View Article Google Scholar -
Demailly
A.,
Moreau
C.,
D. Devos,
Effectiveness of continuous dopaminergic therapies in Parkinson’s disease: A review of L-DOPA pharmacokinetics/pharmacodynamics. Journal of Parkinson’s Disease.
2024;
14
(5)
:
925-39
.
View Article Google Scholar -
Klæstrup
I.H.,
Just
M.K.,
Holm
K.L.,
Alstrup
A.K.O.,
Romero-Ramos
M.,
Borghammer
P.,
N. Van Den Berge,
Impact of aging on animal models of Parkinson's disease. Frontiers in aging neuroscience.
2022;
14
:
909273
.
View Article Google Scholar -
Demir
E.A.,
Karagoz
M.J.E.A.R.,
Platelet-rich plasma (PRP) is a potential self-sourced cognition booster in elderly mice. Experimental Aging Research.
2020;
46
(2)
:
139-53
.
View Article Google Scholar -
Fujino
T.,
Hossain
M.S.,
Mawatari
S.J.P.B.E.M.,
Therapeutic efficacy of plasmalogens for Alzheimer’s disease, mild cognitive impairment, and Parkinson’s disease in conjunction with a new hypothesis for the etiology of Alzheimer’s disease. Peroxisome biology: experimental models, peroxisomal disorders and neurological diseases.
2020;
2020
:
195-212
.
View Article Google Scholar -
Zhao
Y.,
Qian
R.,
Zhang
J.,
Liu
F.,
Iqbal
K.,
Dai
C.-L.,
pathologies and ameliorates cognitive impairment in 3× Tg-AD mice. Alzheimer's research & therapy.
2020;
70
:
1-3
.
View Article Google Scholar -
Charvin
D.,
Medori
R.,
Hauser
R.A.,
Rascol
O.J.N.R.D.D.,
Therapeutic strategies for Parkinson disease: beyond dopaminergic drugs. Nature Reviews Drug Discovery.
2018;
17
(11)
:
804-22
.
View Article Google Scholar -
Wijeyekoon
R.,
Cell replacement therapy for Parkinson's disease. Biochimica et Biophysica Acta (BBA)-Molecular Basis of Disease.
2009;
1792
(7)
:
688-702
.
View Article Google Scholar -
Sanchez-Pernaute
R.,
Lee
H.,
Patterson
M.,
Reske-Nielsen
C.,
Yoshizaki
T.,
Sonntag
K.C.,
Parthenogenetic dopamine neurons from primate embryonic stem cells restore function in experimental Parkinson's disease. Brain.
2008;
131
(Pt 8)
:
2127-39
.
View Article PubMed Google Scholar -
Barker
R.A.,
Widner
H.J.N.,
Immune problems in central nervous system cell therapy. NeuroRx.
2004;
1
:
472-81
.
View Article Google Scholar -
Stoker
T.B.,
Stem Cell Treatments for Parkinson’s Disease. Codon Publications, Brisbane (AU) 2019.
Google Scholar -
Olanow
C.W.,
Obeso
J.A.,
Stocchi
F.J.T.L.N.,
Continuous dopamine-receptor treatment of Parkinson's disease: scientific rationale and clinical implications. The Lancet Neurology.
2006;
5
(8)
:
677-87
.
View Article Google Scholar -
RAJNm
undefined Barker,
Designing stem-cell-based dopamine cell replacement trials for Parkinson’s disease. Nature Medicine.
2019;
25
(7)
:
1045-53
.
View Article Google Scholar -
Chen
W.,
Huang
Q.,
Ma
S.,
Li
M.J.A.C.N.,
Progress in dopaminergic cell replacement and regenerative strategies for Parkinson’s disease. ACS Chemical Neuroscience.
2018;
10
(2)
:
839-51
.
View Article Google Scholar -
Barker
R.A.,
Drouin-Ouellet
J.,
Parmar
M.J.N.R.N.,
Cell-based therapies for Parkinson disease—past insights and future potential. Nature Reviews Neurology.
2015;
11
(9)
:
492-503
.
View Article Google Scholar -
Kusena
J.W.,
Thomas
R.J.,
McCall
M.J.,
Wilson
S.L.J.R.M.,
From protocol to product: ventral midbrain dopaminergic neuron differentiation for the treatment of Parkinson’s disease. Regenerative Medicine.
2019;
14
(11)
:
1057-69
.
View Article Google Scholar -
D. Bhartiya,
Ideal Stem Cell Candidate for Regenerative Medicine: Pluripotent Stem Cells, Adult Stem Cells, or Pluripotent Stem Cells in Adult Organs?. Regenerative Medicine: Laboratory to Clinic. 2017:143-58 2017.
Google Scholar -
Suman
S.,
Domingues
A.,
Ratajczak
J.,
Ratajczak
M.Z.,
.
Potential Clinical Applications of Stem Cells in Regenerative Medicine. In: Ratajczak, M. (eds) Stem Cells. Advances in Experimental Medicine and Biology, vol 1201. Springer, Cham. https://doi.org/10.1007/978-3-030-31206-0_1.
.
View Article Google Scholar -
Zhang
L.,
Zheng
Y.,
Xie
J.,
Potassium channels and their emerging role in parkinson's disease. Brain research bulletin.
2020;
160
:
1-7
.
View Article Google Scholar -
Doi
D.,
Magotani
H.,
Kikuchi
T.,
Ikeda
M.,
Hiramatsu
S.,
Yoshida
K.,
Pre-clinical study of induced pluripotent stem cell-derived dopaminergic progenitor cells for Parkinson’s disease. Nature communications.
2020;
11
(1)
:
3369
.
View Article Google Scholar -
Feigin
V.L.,
Nichols
E.,
Alam
T.,
Bannick
M.S.,
Beghi
E.,
Blake
N.,
Global, regional, and national burden of neurological disorders, 1990–2016: a systematic analysis for the Global Burden of Disease Study 2016. The Lancet Neurology.
2019;
18
(5)
:
459-80
.
View Article Google Scholar -
Schönenberg
A.,
Measuring quality of life with the Parkinson’s Disease Questionnaire-39 in people with cognitive impairment. Plos one.
2022;
17
(4)
:
e0266140
.
View Article Google Scholar -
Cui
X.,
W. Taiyi,
Exploring the mechanism of acupuncture for Parkinson's disease based on network pharmacology techniques. Highlights in Science Engineering and Technology.
2023;
45
:
361-369
.
View Article Google Scholar -
Kwon
S.,
Seo
B.K.,
Kim
S.,
Acupuncture points for treating Parkinson's disease based on animal studies. Chinese Journal of Integrative Medicine.
2016;
22
(10)
:
723-7
.
View Article PubMed Google Scholar -
Zhao
Y.,
Zhang
Z.,
Qin
S.,
Fan
W.,
Li
W.,
Liu
J.,
Acupuncture for Parkinson's Disease: Efficacy Evaluation and Mechanisms in the Dopaminergic Neural Circuit. Neural Plasticity.
2021;
2021
:
9926445
.
View Article PubMed Google Scholar -
Gonzalez
R.,
Garitaonandia
I.,
Poustovoitov
M.,
Abramihina
T.,
McEntire
C.,
Culp
B.,
Neural stem cells derived from human parthenogenetic stem cells engraft and promote recovery in a nonhuman primate model of Parkinson's disease. Cell transplantation.
2016;
25
(11)
:
1945-66
.
View Article Google Scholar -
Li
C.J.P.R.,
Strengthening regulations, recent advances and remaining barriers in stem cell clinical translation in China: 2015–2021 in review. Pharmacological Research.
2022;
182
:
106304
.
View Article Google Scholar -
Muthuraman
M.,
Koirala
N.,
Ciolac
D.,
Pintea
B.,
Glaser
M.,
Groppa
S.,
Deep brain stimulation and L-DOPA therapy: concepts of action and clinical applications in Parkinson's disease. Frontiers in neurology.
2018;
9
:
711
.
View Article Google Scholar -
Zhong
R.,
Huang
W.,
Chen
C.,
Zhang
B.,
Pu
J.,
Zhang
F.J.A.,
Advances in neural stem cells in aging and age-related neurodegenerative diseases. Ageing and Neurodegenerative Diseases.
2025;
5
(2)
.
View Article Google Scholar -
Loewenbrück
K.,
Stem cell-based therapies in Parkinson’s disease: future hope or current treatment option?. Journal of neurology.
2011;
258
(53)
:
346-53
.
View Article Google Scholar -
Li
W.,
Chen
S.,
Human induced pluripotent stem cells in Parkinson's disease: A novel cell source of cell therapy and disease modeling. Progress in Neurobiology.
2015;
134
:
161-77
.
View Article Google Scholar -
Venkataramana
N.K.,
Kumar
S.K.,
Balaraju
S.,
Radhakrishnan
R.C.,
Bansal
A.,
Dixit
A.,
Open-labeled study of unilateral autologous bone-marrow-derived mesenchymal stem cell transplantation in Parkinson's disease. Translational Research. .
2010;
155
(2)
:
62-70
.
View Article Google Scholar -
Wakeman
D.R.,
Dodiya
H.B.,
J.H. Kordower,
Cell transplantation and gene therapy in Parkinson's disease. Mount Sinai Journal of Medicine: A Journal of Translational and Personalized Medicine.
2011;
78
(1)
:
126-58
.
View Article Google Scholar -
Pantcheva
P.,
Reyes
S.,
Hoover
J.,
Kaelber
S.,
Treating non-motor symptoms of Parkinson’s disease with transplantation of stem cells. Expert review of neurotherapeutics.
2015;
15
(10)
:
1231-40
.
View Article Google Scholar -
Daadi
E.W.,
Daadi
E.S.,
Oh
T.,
Li
M.,
Kim
J.,
Daadi
M.M.J.E.N.,
Combining physical & cognitive training with iPSC-derived dopaminergic neuron transplantation promotes graft integration & better functional outcome in parkinsonian marmosets. Experimental Neurology.
2024;
374
:
114694
.
View Article Google Scholar -
Zamanian
M.Y.,
Nazifi
M.,
Khachatryan
L.G.,
Taheri
N.,
Ivraghi
M.S.,
Menon
S.V.,
The neuroprotective effects of agmatine on Parkinson’s disease: Focus on oxidative stress, inflammation and molecular mechanisms. Inflammation.
2024;
:
Online ahead of print
.
View Article PubMed Google Scholar
Comments
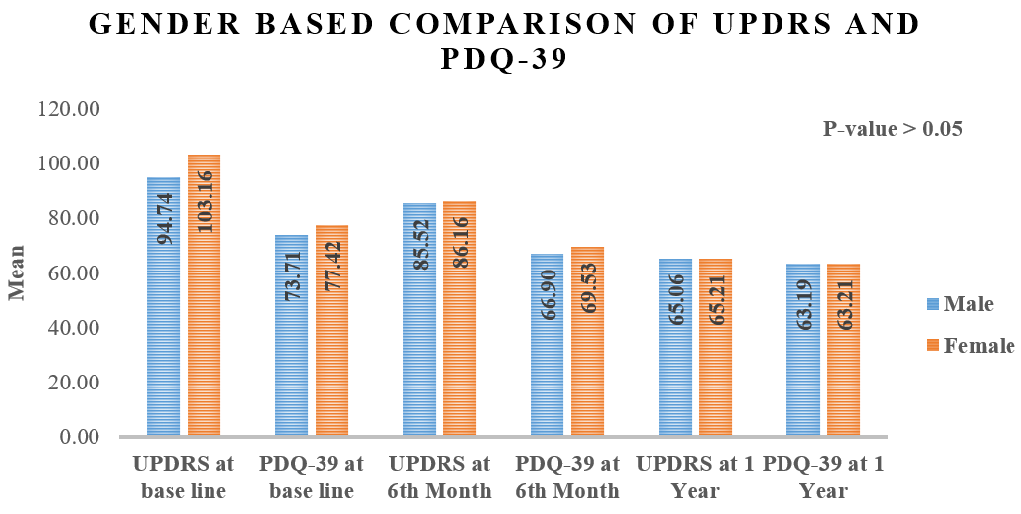
Article Details
Volume & Issue : Vol 12 No 3 (2025)
Page No.: 7236-7245
Published on: 2025-03-31
Citations
Copyrights & License

This work is licensed under a Creative Commons Attribution 4.0 International License.
Search Panel
Pubmed
Google Scholar
Pubmed
Google Scholar
Pubmed
Google Scholar
Pubmed
Google Scholar
Pubmed
Google Scholar
Pubmed
Google Scholar
Pubmed
Google Scholar
Pubmed
Google Scholar
Pubmed
Google Scholar
Pubmed
Google Scholar
Pubmed
Google Scholar
Pubmed
Google Scholar
Pubmed
Google Scholar
Pubmed
Search for this article in:
Google Scholar
Researchgate
- HTML viewed - 720 times
- PDF downloaded - 189 times
- XML downloaded - 50 times