Abstract
Introduction: Oxidative stress (OS) contributes to platelet storage lesion (PSL) and can be attenuated by using antioxidant additives in the storage solution. N-acetyl cysteine (NAC), a thiolcontaining antioxidant, scavenges reactive oxygen species (ROS) directly, upregulates antioxidant defenses, and has anti-apoptotic properties. This study explores the effect of the antioxidant additive NAC on platelets during storage.
Methods: Platelets obtained from the blood of male Wistar rats (n = 5) were resuspended in SSP+ and divided into i) Controls, ii) 0.5-NAC, and iii) 1-NAC; stored at 22◦C for 11 days. The markers of platelet function (aggregation, ATP secretion, P-selectin), viability (caspase-3, MTT), OS (superoxides, nitrites), lipid peroxidation (thiobarbituric reactive substances), protein oxidation (protein sulfhydryls, advanced oxidation protein products), antioxidant defenses (superoxide dismutase, catalase, glutathione, total antioxidant capacity), and metabolism (pH, glucose, lactate dehydrogenase) were analyzed on storage days 1, 4, 7, and 11.
Results: 1- NAC augmented antioxidant defenses, improved viability, and decreased protein oxidation, lipid peroxidation, and platelet activation. Aggregation without collagen decreased in 0.5-NAC and 1- NAC compared to controls. Both 0.5-NAC and 1-NAC were effective in maintaining platelet functions during storage. However, 1-NAC protected platelets from oxidation, enhanced antioxidant defenses, and maintained viability until day 7 of storage. 1-NAC could effectively scavenge ROS and enhance GSH and antioxidant enzymes in comparison to 0.5-NAC.
Conclusion: N-acetyl cysteine at 1 mM concentration in SSP+ could maintain the efficacy of platelets as these cells could endure OS until day 7 of storage. This study emphasizes the potential of NAC as an effective component of platelet storage solutions to prolong the shelf-life of platelets.
Introduction
Platelets stored in plasma beyond 5 days develop lesions, rendering them unsuitable for transfusion1. Platelet additive solutions (PAS), developed during the 1980s2, 3, have aided in reducing the ratio of plasma content, thereby mitigating storage lesions and prolonging the shelf life of stored platelets1. SSP+ or PAS-E (69 mM sodium chloride, 5 mM potassium chloride, 6.7 mM sodium dihydrogen phosphate, 21.5 mM disodium hydrogen phosphate, 1.5 mM magnesium chloride, 30 mM sodium acetate, 11 mM trisodium citrate; pH – 7.2) has proven to maintain the in vitro quality of stored platelets and has extended their shelf life to 9 days4, 5. The potency of PAS in improving viability is still unclear, although there are promising results in terms of platelet function and quality. This limitation can be minimized by optimizing the formulations of PAS6.
Oxidative stress (OS) is a major causative factor of storage lesions. Antioxidant additives such as resveratrol7, 8 , L-carnitine9, 10 , and trehalose11 have proven to alleviate OS and improve the efficacy of stored platelets. N-acetyl cysteine (NAC) is a thiol-containing antioxidant that acts as a glutathione precursor10 and upregulates enzymatic antioxidant defenses12. It has anti-apoptotic properties and reduces platelet activation due to its ability to directly scavenge reactive oxygen species (ROS)13. There are only two studies that have focused on the effect of NAC on platelet function and quality during cold storage. Handigund et al. reported that NAC at a 50 mM concentration could reduce platelet activation and metabolic activity in human platelets during cold storage for 5 days14. However, 50 mM NAC, being a much higher concentration, could affect platelets through the alkalization of pH. Hegde et al. revealed that ROS generated during prolonged cold storage of human platelets was inhibited by 1 mM NAC. Moreover, NAC could increase platelet recovery by preventing its clearance in thrombocytopenic mice post-transfusion15. Hosseini et al. studied only activation, apoptosis, and viability in human platelets stored in PAS-E with NAC for 7 days at 22-24°C16. There are limited studies that evaluate the effects of NAC on oxidative stress and antioxidant defenses in platelets during storage at 22°C. The impact of NAC on redox status and antioxidant defense mechanisms needs to be investigated. Wistar rats were used for this study in order to gain insights into the mechanisms of platelet physiology. The ultrastructural cellular features of rat and human platelets are similar. The receptors on the platelet membrane are similar in both; however, there might be minimal variations in their responses due to distribution differences in rat platelets17.
Thus, this study aims to assess the oxidative modulations influenced by NAC on rat platelets stored in SSP+ (PAS-E). This is the first study to investigate the interaction between OS and antioxidant defenses in response to NAC during platelet storage.
Methods
This study was conducted in accordance with the regulations of the institutional ethical committee (1810/PO/RcBiBt/S/15/CPCSEA).
Chemicals
N-acetyl cysteine and 5,5′-dithiobis-2-nitrobenzoic acid (DTNB) were purchased from Sigma-Aldrich (Mumbai, India). Collagen and Cytochrome C were obtained from SRL Chemicals (Mumbai, India). The 3-(4,5-dimethylthiazol-2-yl)-2,5-diphenyltetrazolium bromide (MTT) reagent was from HiMedia (Mumbai, India). P-selectin (Cat no. KLR2228) and Caspase-3 (Cat no. KLR1648) kits were obtained from KRISHGEN Biosystems (Mumbai, India). The glucose kit was from AutoSpan Gold kit, Arkray (Mumbai, India). All other chemicals used were of analytical grade, and the organic solvents were of spectral grade.
Experimental Design
Blood was collected from male Wistar rats (4-month-old) and platelets were isolated and resuspended in SSP+. Platelets were divided into 3 groups (n = 5): i) Controls: SSP+, ii) 0.5-NAC: 0.5 mM NAC in SSP+, and iii) 1-NAC: 1 mM NAC in SSP+; and stored in polypropylene tubes at 22°C for a period of 11 days. Markers of platelet functions, viability, metabolism, oxidative stress, and antioxidant defenses were analyzed on days 1, 4, 7, and 11 of storage.
Blood Sampling and Platelet Isolation
Male Wistar rats (4 months old) were anesthetized and restrained in dorsal recumbency. Blood from male Wistar rats (4 months old) was carefully aspirated from the heart into collection tubes with CPDA-1 (citrate phosphate dextrose adenine)18 and centrifuged at 1500 rpm for 15 minutes at room temperature. The platelet-rich plasma obtained was then centrifuged at 4000 rpm for 15 minutes at 22°C19. The resulting platelet pellet was gently resuspended in SSP+ (pH 7.4).
Platelet Function and Viability
Platelet Aggregation: Platelets were incubated with and without collagen (2.0 µg/ml) at 37°C. The absorbance was measured at 405 nm20.
ATP Secretion: Platelets were incubated at 37°C with collagen (2.0 µg/ml) and treated with 1.2 M perchloric acid. The absorbance was read at 260 nm and the amount of adenine nucleotides was calculated using an ATP standard21.
Platelet Activation (P-selectin): P-selectin (marker of activation) in the stored platelets was measured quantitatively by the sandwich ELISA method using the Rat P-selectin GENLISATM ELISA kit according to the manufacturer’s instructions. The absorbance was measured at 450 nm.
Platelet Apoptosis (Caspase-3): Caspase-3 (marker of apoptosis) in the platelets was measured quantitatively by the sandwich ELISA method using the Rat CASP3 GENLISATM ELISA kit according to the manufacturer’s instructions. The absorbance was measured at 450 nm.
Cell Viability: Platelets along with the MTT reagent (0.5 mg/ml) were incubated for 4 hours at 37°C. Dimethyl sulfoxide was added and incubated overnight at 37°C. Absorbance was measured at 570 nm22.
Protein: Protein concentration in platelets was determined according to Lowry et al.23
Antioxidant status
Superoxide Dismutase (SOD, EC 1.15.1.1): Carbonate buffer (0.05 M; pH 10.2; 0.1 mM EDTA) was added to platelets, followed by epinephrine, and the absorbance was measured at 480 nm. The SOD activity was expressed as the amount of enzyme that inhibits oxidation of epinephrine by 50%, which is equal to 1 unit24.
Catalase (CAT, EC 1.11.1.6): Platelets were treated with absolute ethanol and incubated in an ice bath for 30 minutes. After incubation, phosphate buffer and 0.066 M hydrogen peroxide were added and the absorbance was measured at 240 nm25. The catalase activity was determined using the molar extinction coefficient 43.6 M cm-1.
Glutathione: Platelets were treated with 4% sulfosalicylic acid, vortexed, and centrifuged at 2500g for 15 minutes. The supernatant was treated with 10 mM DTNB and the absorbance was read at 412 nm26.
Total Antioxidant Capacity (TAC): Platelets were treated with bathocuproinedisulfonic acid disodium salt (0.25 mM) and absorbance was measured at 490 nm. The CuSO4 solution (0.5 mM) and EDTA solution (0.01 M) were added and the final absorbance was measured27. Uric acid was used as the standard.
Lipid peroxidation
Thiobarbituric Acid Reactive Substances (TBARS): Platelets treated with 20% cold trichloroacetic acid in 0.6 M HCl were centrifuged and 0.12 M thiobarbituric acid was added to the supernatant. The samples were incubated in a boiling water bath and the absorbance was read at 532 nm28.
Protein oxidation
Advanced Oxidation Protein Products (AOPP): Platelets were treated with isotonic phosphate buffer, 1.16 M potassium iodide, and glacial acetic acid. The absorbance was measured at 340 nm29. The amount of AOPP was estimated using an extinction coefficient of 26 mM-1 cm-1.
Protein Sulfhydryls: Platelets were treated with sodium phosphate buffer (0.08 M) containing sodium ethylenediaminetetraacetic acid (Na2-EDTA) (0.5 mg/mL) and sodium dodecyl sulfate (2%). DTNB was added and the color developed was measured at 412 nm30. The amount of sulfhydryls was calculated using the molar absorptivity of 13,600 M-1 cm-1.
Oxidative stress
Superoxides: Platelets were incubated with 200 µl cytochrome C (160 µM) at 37°C. The cytochrome C reduction was measured spectrophotometrically at 550 nm31.
Nitrites: Platelets were treated with Griess reagent and incubated in the dark at room temperature. The absorbance was measured at 548 nm. Sodium nitrite was used as the standard to determine the amounts of nitrites32.
Platelet metabolism
pH: The pH in the platelet samples was determined using Fisher Scientific pH strips33.
Glucose: Glucose in the platelets was measured by the glucose oxidase-peroxidase (GOD-POD) method according to the manufacturer’s instructions. The absorbance was measured at 546 nm34.
Lactate Dehydrogenase (LDH, EC 1.1.1.27): Platelets were treated with a mixture of Reagent 1 (Tris, NaOH, and pyruvate) and Reagent 2 (Nicotinamide adenine dinucleotide) and incubated at 37°C. The absorbance was measured at 340 nm35.
Statistical Analyses
The results are represented as Mean ± standard error (SE) (n = 5). Two-way analysis of variance (ANOVA) was performed between the groups (storage days) and sub-groups (between the controls and antioxidant groups) followed by Bonferroni post hoc test using GraphPad Prism-8 Software. The results were considered significant at p < 0.05. Pearson’s correlation was also calculated.
Results
Results are depicted as i) variations between the storage days (groups) and ii) variations between the controls, 0.5-NAC, and 1-NAC (sub-groups) on a particular day of storage.
Platelet Function Markers
Aggregation
With collagen: The variations were similar during storage with respect to day 1 in controls, 0.5-NAC, and 1-NAC (Figure 1 A). Aggregation was similar on each storage day between controls, 0.5-NAC, and 1-NAC.
Without collagen: Aggregation increased by 130% (p < 0.01) on day 4 and 250% (p < 0.0001) on day 7 compared to day 1 and normalized to day 4 levels on day 11 in controls. Aggregation was maintained throughout storage in 0.5-NAC and 1-NAC. Aggregation decreased by 50% (p < 0.0001) in 0.5-NAC and 1-NAC compared to controls on day 7 (Figure 1 B).
ATP Secretion
ATP secretion peaked on day 4 by 70% (p < 0.05) and further decreased by 50% (p < 0.01) on days 7 and 11 in controls. ATP secretion declined by approximately 45% on day 7 (p < 0.01) and day 11 (p < 0.05) in 0.5-NAC, whereas it decreased by 50% (p < 0.05) on day 7 in 1-NAC with respect to day 4 (Figure 1C). ATP secretion was similar on each storage day between controls, 0.5-NAC, and 1-NAC.
Platelet Activation (P-selectin)
P-selectin peaked on day 4 by 150% (p < 0.0001) and reduced by approximately 65% (p < 0.0001) on days 7 and 11 in controls. 0.5-NAC showed increments of 125% (p < 0.0001) on day 4 and declined by approximately 60% (p < 0.0001) on days 7 and 11. P-selectin was maintained throughout storage in 1-NAC. P-selectin declined by 80% (p<0.0001) in 1-NAC compared to controls and 0.5-NAC on day 4 (Figure 1D).
Platelet Viability and Quality
Platelet Apoptosis (Caspase-3)
The variations in Caspase-3 levels were similar during storage with respect to day 1 in controls, 0.5-NAC, and 1-NAC. Caspase-3 decreased by approximately 60% (p < 0.01) in 1-NAC compared to 0.5-NAC (on days 1, 4, 7) and controls (on day 4) (Figure 1E).
Cell Viability
Controls exhibited decrements of approximately 55% (p < 0.05) and approximately 85% (p < 0.0001) on days 7 and 11 respectively, compared to days 1 and 4. Viable platelets decreased by 50% (p < 0.001) and 90% (p < 0.0001) on days 7 and 11 compared to day 1 in 0.5-NAC. 1-NAC showed decrements of 30% (p < 0.05) on days 4 and 7 and 75% (p < 0.0001) on day 11 compared to day 1 in 1-NAC (Figure 1 F). The variations in platelet viability were similar on each storage day between controls, 0.5-NAC, and 1-NAC.
Antioxidant Defenses
Superoxide Dismutase (SOD)
SOD increased by 1-fold (p < 0.05) on day 4 compared to day 1 and further decreased by approximately 75% (p < 0.01) on days 7 and 11 in controls. SOD declined by approximately 88% (p < 0.05) on days 7 and 11 compared to day 1 in 0.5-NAC. 1-NAC showed elevations of 7-fold (p < 0.0001) on day 4 compared to day 1 which further declined by approximately 85% (p< 0.001) on days 7 and 11. 1-NAC exhibited increments of 1-fold and 3-fold (p < 0.0001) compared to controls and 0.5-NAC, respectively, on day 4 (Figure 2A).
Catalase
Variations in catalase were similar during storage with respect to day 1 in controls (3.72 U×10-4/ mg protein) and 0.5-NAC (6.85 U×10-4/ mg protein). Catalase remained constant at approximately 4.15 U×10-4/ mg protein until day 7 and decreased by 45% on day 11 in 1-NAC.
Glutathione (GSH)
GSH increased by 4-fold (p < 0.0001) on day 4 compared to day 1 and further decreased by approximately 50% (p < 0.05) on days 7 and 11 in controls. GSH increased by 125% on day 4 and normalized on days 7 and 11 to day 1 levels. 1-NAC exhibited increments of 1-fold (p < 0.0001) on day 4 compared to day 1 and further declined by 65% (p < 0.0001) on days 7 and 11. 1-NAC exhibited increments of 1-fold (p < 0.0001) compared to controls and 0.5-NAC, respectively, on day 4 (Figure 2B).
Total Antioxidant Capacity (TAC)
TAC was maintained throughout storage in controls. TAC peaked by 40% in 0.5-NAC and by 50% in 1-NAC on day 7 compared to day 1 and normalized to day 4 levels on day 11, respectively. TAC increased by 60% (p < 0.01) in 1-NAC compared to controls on day 7 (Figure 2C).
Protein Oxidation
Advanced Oxidation Protein Products (AOPP)
AOPP peaked on day 11 by 60% (p < 0.05) compared to days 1 and 4 in controls. AOPP decreased by approximately 45% on day 4 and further increased by approximately 200% (p < 0.05) on day 11 in 0.5-NAC, whereas it was maintained in 1-NAC throughout storage. AOPP increased by 1-fold (p < 0.05) in 1-NAC compared to 0.5-NAC on day 4 (Figure 3A).
Protein Sulfhydryls (SH)
SH decreased by 65% (p < 0.05) on day 4 compared to day 1 and normalized on days 7 and 11 to day 1 levels in controls. 0.5-NAC showed decrements of approximately 60% (p < 0.01) on days 4, 7, and 11 compared to day 1, whereas SH increased by 1-fold (p < 0.01) on day 4 compared to day 1 and further declined by approximately 35% on days 7 and 11 in 1-NAC. 1-NAC exhibited increments of 3-fold and 1-fold (p < 0.001) compared to controls and 0.5-NAC, respectively, on day 4 (Figure 3B).
Lipid Peroxidation
Thiobarbituric Acid Reactive Substances (TBARS)
TBARS decreased by 55% (p < 0.001) on day 11 compared to day 1 in controls. The variations in TBARS were similar to day 1 in 0.5-NAC, whereas it declined by 75% (p < 0.05) on day 11 compared to day 1 in 1-NAC (Figure 3C). TBARS were maintained on each storage day between controls, 0.5-NAC, and 1-NAC.
Oxidative Stress
Superoxides
Variations in superoxides were similar to day 1 (0.107 ± 0.03 mM mg/protein) in controls. Superoxides remained constant until day 7 and declined by 30% on day 11 compared to day 1 (0.201 ± 0.02 mM mg/protein) in 0.5-NAC. Superoxides peaked on day 4 by 1-fold, normalized to day 1 levels (0.04 ± 0.007 mM mg/protein) on day 7, and increased by 1-fold on day 11 in 1-NAC. Superoxides declined by 75% (p < 0.01) in 1-NAC compared to 0.5-NAC (0.201 ± 0.04 mM mg/protein) on day 7.
Nitrites
Nitrites peaked on day 7 by 4-fold (p < 0.0001) and normalized on day 11 to day 1 levels (0.2 ± 0.07 mM/mg protein) in controls. Nitrites increased by 68% on day 4, normalized on day 7 to day 1 levels (0.446 ± 0.04 mM/mg protein), and further declined by 70% on day 11 in 0.5-NAC. 1-NAC exhibited elevations of 10-fold on day 4 and normalized on day 11 to day 1 levels (0.038 ± 0.005 mM/mg protein). Nitrites declined by 60% (p < 0.05) in 1-NAC compared to controls (1.03 ± 0.25 mM/mg protein) on day 7.
Storage period (days) | Sub-groups | Glucose (mM/ L) | Lactate dehydrogenase (U*10 -6 / mg protein) |
1 | Control | 14.86 ± 2.12 | 28.88 ± 6.28 |
0.5-NAC | 18.03 ± 1.3 | 16.68 ± 2.72 | |
1-NAC | 12.33 ± 4.2 | 14.55 ± 2.44 | |
4 | Control | 0.55 ± 0.07* | 17.04 ± 4.88 |
0.5-NAC | 1.27 ± 0.35* | 9.68 ± 2.30* | |
1-NAC | 1.68 ± 0.14* | 26.7 ± 2.60 | |
7 | Control | 1.55 ± 0.25* | 10.46 ± 1.47 |
0.5-NAC | 1.31 ± 0.2* | 13.04 ± 3.32 | |
1-NAC | 1.35 ± 0.35* | 8.23 ± 2.12 | |
11 | Control | 2.62 ± 0.46* | 24.0 ± 5.58 |
0.5-NAC | 1.4 ± 0.23* | 8.21 ± 0.72* | |
1-NAC | 1.90 ± 0.2* | 19.4 ± 3.03 |
Metabolic Markers
pH
The changes in pH during storage were similar during storage in all the sub-groups. pH decreased from 7 on day 1 to 6.6 on day 11 in controls, whereas it was maintained at approximately 7 in 0.5-NAC and 1-NAC throughout storage.
Glucose
Glucose significantly decreased on days 4, 7, and 11 in all the subgroups - controls by approximately 90% (p < 0.0001), 0.5-NAC by approximately 90% (p < 0.0001), and 1-NAC by approximately 85% (p < 0.001) compared to day 1 (Table 1). Glucose was maintained on each storage day between controls, 0.5, and 1-NAC.
Lactate Dehydrogenase (LDH)
LDH decreased by 65% on day 7 and normalized on day 11 to day 1 levels in controls. 0.5-NAC exhibited decrements of 75% (p < 0.05) on day 4 and 68% (p < 0.01) on day 11 compared to day 1. LDH increased on day 4 by 140% and normalized on day 11 to day 1 levels in 1-NAC (Table 1). The variations in LDH were similar on each storage day between controls, 0.5, and 1-NAC.
To summarize, 0.5- and 1-NAC could preserve platelet function compared to controls by reducing P-selectin and aggregation without collagen. However, 1-NAC could augment protein sulfhydryls and antioxidant defenses such as SOD and GSH and lower superoxide levels, thereby increasing the total antioxidant capacity compared to controls and 0.5-NAC. 1-NAC could maintain the viability of platelets until day 7 as observed in caspase levels.
Discussion
Storage lesions lead to diminished platelet function, thereby affecting the quality of stored platelets. Antioxidant additives can be beneficial, as oxidative stress (OS) overwhelms the endogenous antioxidant defenses of platelets during storage. N-acetylcysteine (NAC), a potential antioxidant, was employed as an additive in SSP+ to explore the response of platelets.
Platelets respond to agonists (such as collagen and thrombin) leading to their activation, causing aggregation and releasing secondary messengers such as ATP, which eventually form a stable clot in vivo36. During storage, premature activation of platelets causes aggregation without the involvement of agonists, thus decreasing their quality. Platelets are activated at higher levels of reactive oxygen species (ROS)37. This was evident in P-selectin (CD62P, a marker of platelet activation), which is proportional to aggregation without an agonist (collagen) on days 4 and 7 in controls. P-selectin was lower in 1-NAC compared to 0.5-NAC, indicating that 1-NAC could scavenge ROS until the end of storage and thereby influence platelet activation. This is in accordance with the results of Hosseini et al., where platelets treated with NAC at a 1 mM concentration for 5 days exhibited lower P-selectin expression16. NAC has an anti-aggregatory effect on platelets, which is evidenced by decreased aggregation without collagen in the antioxidant groups on day 7 compared to controls. ATP released during activation is converted by adenylate cyclase to cAMP, which is required for platelet functional responses. Low ATP levels facilitate aggregation responses38. Thus, aggregation in response to collagen was maintained during storage in all groups due to lower ATP levels.
Apoptosis induced by OS is one of the key factors for platelet storage lesions. NAC prevents apoptosis by modulating ROS such as superoxides (O2˙) and hydrogen peroxide (H2O2)39. Caspase-3, a marker of apoptosis, and superoxides were maintained in 1-NAC (r = 0.728) due to the scavenging effect of NAC. Caspase-3 levels were lower in 1-NAC than in controls and 0.5-NAC. This was also evidenced by higher viability in 1-NAC (Caspase-3 vs. cell viability in 1-NAC, r = -0.7).
NAC scavenges ROS such as hydrogen peroxide (H2O2), superoxide (O2˙), hydroxyl (˙OH), and nitrite (˙NO2) radicals directly; and upregulates antioxidant enzymes such as SOD, CAT, and glutathione peroxidase (GPx). Also, NAC acts as a precursor of GSH by providing cysteine moieties, leading to increased GSH levels13, 40. This was evident in the results of antioxidant defenses in 1-NAC. 1-NAC enhanced SOD and GSH on day 4. Antioxidant enzymes significantly increased on day 4, resulting in decrements in ROS, leading to lower SOD and GSH levels on days 7 and 11. The scavenging activity of 1-NAC is reflected in superoxide and nitrite levels, as they decreased on day 7 compared to controls. This also resulted in higher total antioxidant capacity on day 7 in 1-NAC. SOD and GSH were maintained in 0.5-NAC, whereas 1-NAC was beneficial as it augmented antioxidant enzymes, thereby delaying the onset of OS.
NAC, being a precursor of GSH, has aided in the replenishment of SH groups as revealed by elevations in SH on day 4 in 1-NAC (GSH vs SH, r = 0.8). AOPP levels were maintained throughout storage. Thus, 1-NAC could protect the proteins from oxidation throughout storage. However, 0.5-NAC was beneficial until day 7 in terms of AOPP, whereas it could not prevent SH from oxidation from day 4. TBARS, an index of lipid peroxidation, significantly decreased in 1-NAC on day 11, whereas it was maintained in 0.5-NAC throughout the storage period. NAC directly scavenges highly reactive hydroxyl radicals and serves as a substrate for the resynthesis of GSH41, 42. GSH plays an important role in protecting membrane lipids, thereby minimizing lipid peroxidation. Hence, 0.5- and 1-NAC have protected the membrane lipids from oxidative attack.
Platelets metabolize glucose to fulfill their energy requirements, which affects the pH leading to cellular damage. Glucose significantly decreased throughout storage in all groups, although the pH was maintained. SSP+ contains acetate, which serves as a secondary metabolite, providing a buffering effect, thereby maintaining the pH and LDH.
Major changes were observed on day 4 of the storage period as shown in the results for P-selectin, SOD, and GSH. Hence, day 4 could be a crucial period in terms of platelet storage and a major indicator of the quality of stored platelets. This was evidenced in the SOD results of controls, as it increased on day 4, which can be attributed to antioxidant defenses in the plasma component of SSP+. However, on day 11, there was a decrease in viable cells, which is reflected in lower P-selectin, SOD, GSH, and TBARS in both controls and experimentals. Thus, NAC in SSP+ could maintain the efficacy of platelets, as these cells could endure OS until day 7 of storage.
This study provides new avenues to enhance the efficacy of stored platelets, develop better storage solutions, and serves as a basis for post-transfusion studies for clinical practice.
Animal models are used due to minimal variations in genetics, age, diet, and microenvironment. Thus, the outcome can be related to the interventions in the experimental design. The present study was conducted on rat platelets in order to gain insights into the mechanisms of platelet physiology. Thus, further post-transfusion studies are required for investigations in human samples.
Conclusions
0.5-NAC and 1-NAC were effective in maintaining platelet functions during storage. However, 1-NAC exhibited greater potential in protecting platelets from oxidative damage, augmenting endogenous antioxidant defenses, and maintaining platelet viability until day 7 of storage. Hence, N-acetyl cysteine at a 1 mM concentration as an additive in SSP+ could maintain the efficacy of platelets and protect them from oxidative modifications. This study emphasizes the potential of NAC as an effective component of platelet storage solutions in prolonging the shelf-life of platelets. Antioxidants as additives have the potential to improve platelet efficacy. Validations with post-transfusion studies can lead to the development of better storage solutions.
Abbreviations
AOPP - Advanced Oxidation Protein Products, ATP - Adenosine Triphosphate, CAT - Catalase, GSH - Glutathione, GPx - Glutathione Peroxidase, LDH - Lactate Dehydrogenase, MTT - 3-(4,5-dimethylthiazol-2-yl)-2,5-diphenyltetrazolium bromide, NAC - N-acetyl cysteine, OS - Oxidative Stress, PAS - Platelet Additive Solutions, PSL - Platelet Storage Lesion, ROS - Reactive Oxygen Species, SH - Protein sulfhydryls, SOD - Superoxide Dismutase, TAC - Total Antioxidant Capacity, TBARS - Thiobarbituric Acid Reactive Substances
Acknowledgments
The authors acknowledge JAIN (Deemed-to-be University) for their support towards this study (Minor research project). The authors thank Central Research Laboratory, Kempegowda Institute of Medical Sciences, Bengaluru, India and Nargund College of Pharmacy, Bengaluru, India for their support. The authors also thank Dr. Leela Iyengar, Dr. Sowmya Ravikumar, Dr. Manasa Mithun, Dr. Carl Hsieh and Ms. M Pallavi and Ms. Dharmavaram Sravani.
Author’s contributions
Magdaline Christina Rajanand: Original draft preparation, formal analysis, investigation and writing; Anusha Berikai Ananthakrishna: Investigation. Dr. Vani Rajashekaraiah: Conceptualization, review, editing, resources and supervision. All authors read and approved the final manuscript.
Funding
This research was supported by minor research grant from JAIN (Deemed-to-be University) (Reference number: JU/MRP/SOS/2022).
Availability of data and materials
Data generated or analyzed during this study are provided in full within the published article.
Ethics approval and consent to participate
This study was conducted in accordance with the animal care guidelines of Committee for the Purpose of Control and Supervision of Experiments on Animals (CPCSEA), Institutional Animal Ethics Committee (IAEC, New Delhi), India. Invasive/ Non-invasive experiments were not conducted on the animals. Only whole blood was drawn from the animals according to the animal care guidelines of CPCSEA (1810/PO/RcBiBt/S/15/CPCSEA).
Consent for publication
Not applicable.
Competing interests
The authors declare that they have no competing interests.
References
-
Rajashekaraiah
V.,
Rajanand
M.C.,
Platelet storage: progress so far. Journal of Thrombosis and Thrombolysis.
2023;
55
(1)
:
9-17
.
View Article PubMed Google Scholar -
Rock
G.,
Swenson
S.D.,
Adams
G.A.,
Platelet storage in a plasma-free medium. Transfusion.
1985;
25
(6)
:
551-6
.
View Article PubMed Google Scholar -
Adams
G.A.,
Swenson
S.D.,
Rock
G.,
Survival and recovery of human platelets stored for five days in a non-plasma medium. Blood.
1986;
67
(3)
:
672-5
.
View Article PubMed Google Scholar -
Hornsey
V.S.,
McColl
K.,
Drummond
O.,
McMillan
L.,
Morrison
A.,
Morrison
L.,
Extended storage of platelets in SSP platelet additive solution. Vox Sanguinis.
2006;
91
(1)
:
41-6
.
View Article PubMed Google Scholar -
Saunders
C.,
Rowe
G.,
Wilkins
K.,
Holme
S.,
Collins
P.,
In vitro storage characteristics of platelet concentrates suspended in 70% SSP+(TM) additive solution versus plasma over a 14-day storage period. Vox Sanguinis.
2011;
101
(2)
:
112-21
.
View Article PubMed Google Scholar -
Alhumaidan
H.,
Sweeney
J.,
Current status of additive solutions for platelets. Journal of Clinical Apheresis.
2012;
27
(2)
:
93-8
.
View Article PubMed Google Scholar -
Ekaney
M.L.,
Gray
G.G.,
McKillop
I.H.,
Evans
S.L.,
Enhanced platelet function in cold stored whole blood supplemented with resveratrol or cytochrome C. The Journal of Trauma and Acute Care Surgery.
2018;
85
(1S)
:
92-7
.
View Article PubMed Google Scholar -
Wang
L.,
Xie
R.,
Fan
Z.,
Yang
J.,
Liang
W.,
Wu
Q.,
The contribution of oxidative stress to platelet senescence during storage. Transfusion.
2019;
59
(7)
:
2389-402
.
View Article PubMed Google Scholar -
Mithun
M.,
Rajashekaraiah
V.,
L-Carnitine as an additive in Tyrode's buffer during platelet storage. Blood Coagulation & Fibrinolysis.
2018;
29
(7)
:
613-21
.
View Article PubMed Google Scholar -
Amiri
F.,
Dahaj
M.M.,
Siasi
N.H.,
Deyhim
M.R.,
Treatment of platelet concentrates with the L-carnitine modulates platelets oxidative stress and platelet apoptosis due to mitochondrial reactive oxygen species reduction and reducing cytochrome C release during storage. Journal of Thrombosis and Thrombolysis.
2021;
51
(2)
:
277-85
.
View Article PubMed Google Scholar -
Wang
X.,
Fan
Y.,
Shi
R.,
Li
J.,
Zhao
S.,
Quality assessment of platelets stored in a modified platelet additive solution with trehalose at low temperature (10 C) and in vivo effects on rabbit model of thrombocytopenia. Platelets.
2015;
26
(1)
:
72-79
.
View Article PubMed Google Scholar -
Zafarullah
M.,
Li
W.Q.,
Sylvester
J.,
Ahmad
M.,
Molecular mechanisms of N-acetylcysteine actions. Cellular and Molecular Life Sciences.
2003;
60
(1)
:
6-20
.
View Article PubMed Google Scholar -
Samuni
Y.,
Goldstein
S.,
Dean
O.M.,
Berk
M.,
The chemistry and biological activities of N-acetylcysteine. Biochimica et Biophysica Acta.
2013;
1830
(8)
:
4117-29
.
View Article PubMed Google Scholar -
Handigund
M.,
Bae
T.W.,
Lee
J.,
Cho
Y.G.,
Evaluation of in vitro storage characteristics of cold stored platelet concentrates with N acetylcysteine (NAC). Transfusion and Apheresis Science : Official Journal of the World Apheresis Association : Official Journal of the European Society for Haemapheresis.
2016;
54
(1)
:
127-38
.
View Article PubMed Google Scholar -
Hegde
S.,
Wellendorf
A.M.,
Zheng
Y.,
Cancelas
J.A.,
Antioxidant prevents clearance of hemostatically competent platelets after long-term cold storage. Transfusion.
2021;
61
(2)
:
557-67
.
View Article PubMed Google Scholar -
Hosseini
E.,
Ghasemzadeh
M.,
Atashibarg
M.,
Haghshenas
M.,
ROS scavenger, N-acetyl-l-cysteine and NOX specific inhibitor, VAS2870 reduce platelets apoptosis while enhancing their viability during storage. Transfusion.
2019;
59
(4)
:
1333-43
.
View Article PubMed Google Scholar -
Takahashi
O.,
Characteristics of rat platelets and relative contributions of platelets and blood coagulation to haemostasis. Food and Chemical Toxicology.
2000;
38
(2-3)
:
203-18
.
View Article PubMed Google Scholar -
Devi
S. Asha,
Subramanyam
M.V.,
Vani
R.,
Jeevaratnam
K.,
Adaptations of the antioxidant system in erythrocytes of trained adult rats: impact of intermittent hypobaric-hypoxia at two altitudes. Comparative Biochemistry and Physiology. Toxicology & Pharmacology : CBP.
2005;
140
(1)
:
59-67
.
View Article PubMed Google Scholar -
Carneiro
A.M.,
Blakely
R.D.,
Serotonin-, protein kinase C-, and Hic-5-associated redistribution of the platelet serotonin transporter. The Journal of Biological Chemistry.
2006;
281
(34)
:
24769-80
.
View Article PubMed Google Scholar -
Born
G.V.,
Cross
M.J.,
The aggregation of blood platelets. The Journal of Physiology.
1963;
168
(1)
:
178-95
.
View Article PubMed Google Scholar -
Wachowicz
B.,
Olas
B.,
Zbikowska
H.M.,
Buczyński
A.,
Generation of reactive oxygen species in blood platelets. Platelets.
2002;
13
(3)
:
175-82
.
View Article PubMed Google Scholar -
Shiri
R.,
Yari
F.,
Ahmadinejad
M.,
Vaeli
S.,
Tabatabaei
M.R.,
The caspase-3 inhibitor (peptide Z-DEVD-FMK) affects the survival and function of platelets in platelet concentrate during storage. Blood Research.
2014;
49
(1)
:
49-53
.
View Article PubMed Google Scholar -
Lowry
O. H.,
Rosebrough
N. J.,
Farr
A. L.,
Randall
R. J.,
Protein measurement with the Folin phenol reagent. The Journal of biological chemistry.
1951;
193
(1)
:
265-275
.
PubMed Google Scholar -
Misra
H.P.,
Fridovich
I.,
The role of superoxide anion in the autoxidation of epinephrine and a simple assay for superoxide dismutase. The Journal of Biological Chemistry.
1972;
247
(10)
:
3170-5
.
View Article PubMed Google Scholar -
Aebi
H.,
Catalase in vitro. Methods in Enzymology.
1984;
105
:
121-6
.
View Article PubMed Google Scholar -
Beutler
E.,
Modified procedure for the estimation reduced glutathione. The Journal of Laboratory and Clinical Medicine.
1963;
61
:
882
.
PubMed Google Scholar -
Cruz
G. Da,
Use of bathocuproine for the evaluation of the antioxidant power in liquids and solutions. 2003; US patent 6613577. 2003
.
-
Olas
B.,
Nowak
P.,
Kolodziejczyk
J.,
Ponczek
M.,
Wachowicz
B.,
Protective effects of resveratrol against oxidative/nitrative modifications of plasma proteins and lipids exposed to peroxynitrite. The Journal of Nutritional Biochemistry.
2006;
17
(2)
:
96-102
.
View Article PubMed Google Scholar -
Witko-Sarsat
V.,
Friedlander
M.,
Capeillère-Blandin
C.,
Nguyen-Khoa
T.,
Nguyen
A.T.,
Zingraff
J.,
Advanced oxidation protein products as a novel marker of oxidative stress in uremia. Kidney International.
1996;
49
(5)
:
1304-13
.
View Article PubMed Google Scholar -
Habeeb
A.F.,
Reaction of protein sulfhydryl groups with Ellman's reagent. Methods in Enzymology.
1972;
25
:
457-64
.
View Article PubMed Google Scholar -
Olas
B.,
Wachowicz
B.,
Resveratrol and vitamin C as antioxidants in blood platelets. Thrombosis Research.
2002;
106
(2)
:
143-8
.
View Article PubMed Google Scholar -
Chen
L.Y.,
Mehta
P.,
Mehta
J.L.,
Oxidized LDL decreases L-arginine uptake and nitric oxide synthase protein expression in human platelets: relevance of the effect of oxidized LDL on platelet function. Circulation.
1996;
93
(9)
:
1740-6
.
View Article PubMed Google Scholar -
Amorini
A.M.,
Tuttobene
M.,
Lazzarino
G.,
Denti
G.,
Evaluation of biochemical parameters in platelet concentrates stored in glucose solution. Blood Transfusion.
2007;
5
(1)
:
24-32
.
View Article PubMed Google Scholar -
Basak
A.,
Development of a rapid and inexpensive plasma glucose estimation by two-point kinetic method based on glucose oxidase-peroxidase enzymes. Indian Journal of Clinical Biochemistry.
2007;
22
(1)
:
156-60
.
View Article PubMed Google Scholar -
Buhl
S.N.,
Jackson
K.Y.,
Optimal conditions and comparison of lactate dehydrogenase catalysis of the lactate-to-pyruvate and pyruvate-to-lactate reactions in human serum at 25, 30, and 37 degrees C. Clinical chemistry.
1978;
24
(5)
:
828-831
.
View Article PubMed Google Scholar -
Born
G.V.,
Feinberg
H.,
Binding of adenosine diphosphate to intact human platelets. The Journal of Physiology.
1975;
251
(3)
:
803-16
.
View Article PubMed Google Scholar -
Ghasemzadeh
M.,
Hosseini
E.,
Platelet granule release is associated with reactive oxygen species generation during platelet storage: A direct link between platelet pro-inflammatory and oxidation states. Thrombosis Research.
2017;
156
:
101-4
.
View Article PubMed Google Scholar -
Henry
R.L.,
Platelet function. Seminars in Thrombosis and Hemostasis.
1977;
4
(2)
:
93-122
.
View Article PubMed Google Scholar -
Lin
K.T.,
Xue
J.Y.,
Sun
F.F.,
Wong
P.Y.,
Reactive oxygen species participate in peroxynitrite-induced apoptosis in HL-60 cells. Biochemical and Biophysical Research Communications.
1997;
230
(1)
:
115-9
.
View Article PubMed Google Scholar -
Zaragoza
A.,
Díez-Fernández
C.,
Alvarez
A.M.,
Andrés
D.,
Cascales
M.,
Effect of N-acetylcysteine and deferoxamine on endogenous antioxidant defense system gene expression in a rat hepatocyte model of cocaine cytotoxicity. Biochimica et Biophysica Acta.
2000;
1496
(2-3)
:
183-95
.
View Article PubMed Google Scholar -
Kerksick
C.,
Willoughby
D.,
The antioxidant role of glutathione and N-acetyl-cysteine supplements and exercise-induced oxidative stress. Journal of the International Society of Sports Nutrition.
2005;
2
(2)
:
38-44
.
View Article PubMed Google Scholar -
Paul
M.,
Thushara
R.M.,
Jagadish
S.,
Zakai
U.I.,
West
R.,
Kemparaju
K.,
Novel sila-amide derivatives of N-acetylcysteine protects platelets from oxidative stress-induced apoptosis. Journal of Thrombosis and Thrombolysis.
2017;
43
(2)
:
209-16
.
View Article PubMed Google Scholar
Comments
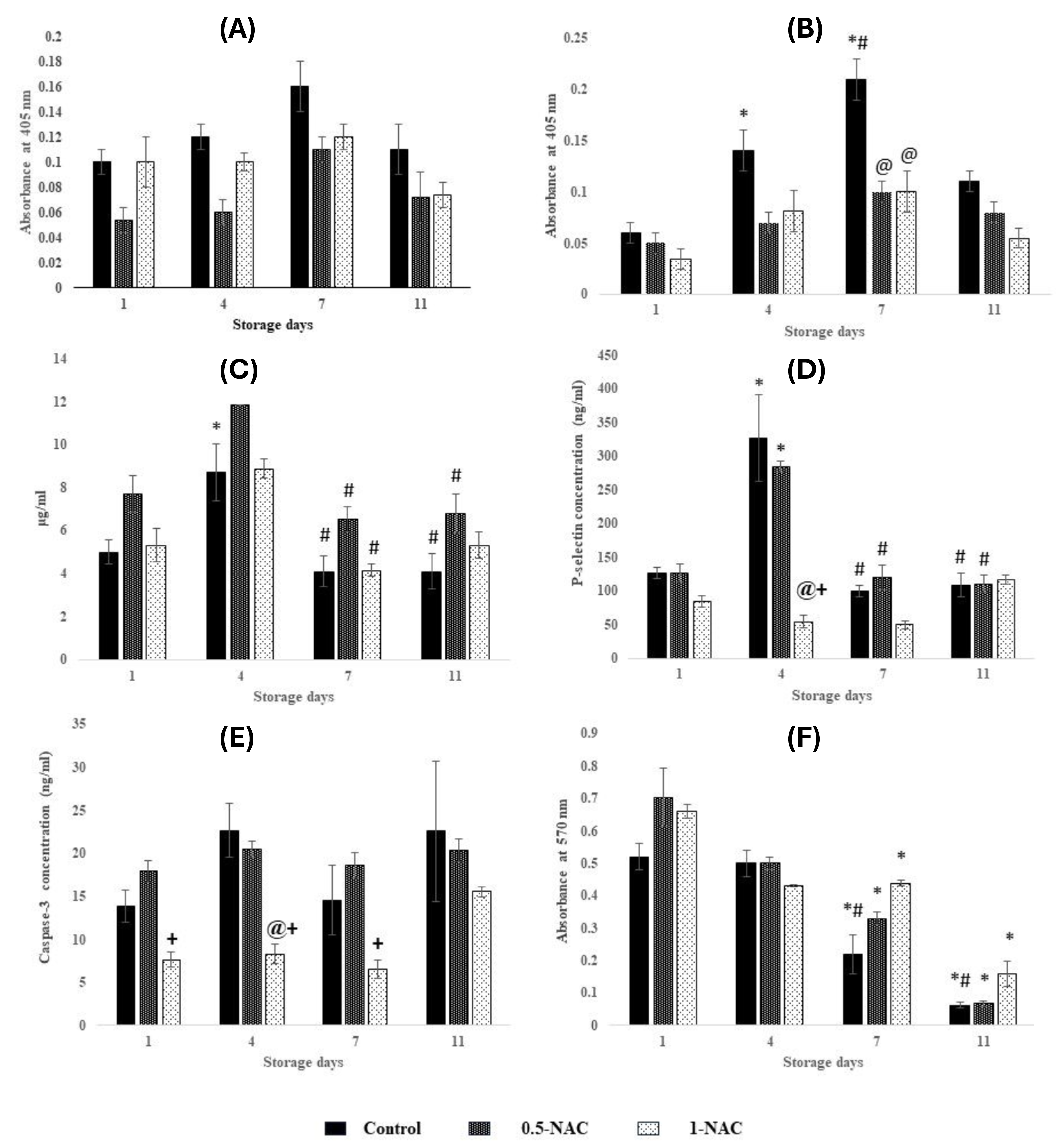
Article Details
Volume & Issue : Vol 11 No 11 (2024)
Page No.: 6950-6959
Published on: 2024-11-30
Citations
Copyrights & License

This work is licensed under a Creative Commons Attribution 4.0 International License.
Search Panel
Pubmed
Google Scholar
Pubmed
Google Scholar
Pubmed
Search for this article in:
Google Scholar
Researchgate
- HTML viewed - 1274 times
- PDF downloaded - 390 times
- XML downloaded - 74 times