Abstract
Background: Platelet-rich plasma (PRP) emerges as a promising therapy, exhibiting noticeable anti-diabetic potential. Nevertheless, the precise underlying mechanism remains enigmatic. In the current investigation, we aimed to scrutinize the potential therapeutic ramifications of PRP in alloxan-induced diabetic mice, with a particular focus on its modulation of glycogen metabolism and glucose transport.
Methods: Forty mice were randomly divided into four groups: NC (Control), PC (PRP treatment), DC (Alloxan dose), and DT (Alloxan+PRP treatment). PRP was administered subcutaneously over four weeks, with a frequency of twice-weekly dosing, to diabetic mice.
Results: Our findings illustrate that PRP maintained glucose homeostasis through its modulatory influence on glycogen metabolism and glucose transporters (Glut2 and Glut4) on the hepatocyte membrane. PRP substantially reduced hepatic glycogen content significantly (P = 0.0148). Moreover, PRP treatment effectively preserved the structural integrity of hepatic lobules and maintained glycogen levels within the cells. There was a substantial increase in glycogen accumulation within the cytoplasm of liver cells in the diabetic group (DC). However, treatment with platelet-rich plasma (PRP) in the diabetes-treated (DT) group resulted in a marked improvement in glycogen accumulation within liver cells.
Conclusion: In aggregate, we concluded that PRP has the potential to ameliorate hyperglycemia in DM by orchestrating the interplay of glycogen metabolism and glucose transport within the liver, ultimately restoring glycogen deposition in hepatic tissues.
Introduction
Diabetes Mellitus (DM) is a global problem characterized by hyperglycemia. It results from insufficient insulin secretion and/or diminished insulin sensitivity1. It arises from the intricate interplay of genetic, epigenetic, and lifestyle factors, including aging, dietary habits, obesity, sedentary living, psychological stress, and urbanization2. Prolonged exposure to hyperglycemia is associated with the emergence of diabetes-related complications, such as nephropathy, neuropathy, retinopathy, and cardiovascular disease3. Furthermore, poorly controlled DM can lead to glycogenic hepatopathy, marked by the excessive accumulation of glycogen within hepatocytes4.
Glycogen synthesis and its degradation are precisely mediated by enzymes and hormonal mechanisms that help adapt glycogen metabolism according to the overall availability of glucose and its demand in the body5. The liver, a major glycogen reservoir, intricately governs glucose release and contributes to the homeostasis of glucose metabolism6. Dysregulation of these physiological processes is a central feature of DM, resulting in hyperglycemia during both postprandial and fasting states7.
Platelet-rich plasma (PRP) is a blood-derived biomaterial that aids in transporting vital growth factors (GFs) and cytokines from platelet granules to specific sites, promoting tissue regeneration8. Platelets, besides their primary role in blood clotting, influence glycogen metabolism through various mechanisms. Upon activation, platelets release growth factors (GFs) that play a complex role in glycogen metabolism and glucose transport in diabetics9. Platelet-derived growth factors (PDGFs) stimulate glycogen synthesis in hepatocytes and muscle cells10. Platelet factor 4 (PF4) inhibits glycogenolysis in the liver and muscle. Transforming growth factor - β (TGF-β) regulates glycogen synthase and glycogen phosphorylase activity11. Moreover, previous studies have highlighted that PDGFs can impact glucose transport by either stimulating the expression of glucose transporters or enhancing glucose uptake in cells12. They also promote insulin receptor substrate 1 (IRS-1) phosphorylation, enhancing insulin signaling13. These GFs are also critically important for tissue regeneration, from hemostasis to tissue remodeling14. Therefore, PRP represents a novel therapeutic avenue for addressing diabetes.
Previous studies have indicated that PRP may exert its anti-diabetic effects by modulating glucose metabolism and the Wnt signaling pathway in the livers of diabetic mice15. Dysregulation of glycogen metabolism in systemic conditions like DM can manifest histologically in the liver, leading to significant hepatic dysfunction. However, it remains to be elucidated whether PRP's anti-diabetic action is mediated by the regulation of glycogen metabolism. This study aims to explore the impact of PRP on hepatic glycogen metabolism and assess its histological deposition in diabetic mice.
Methods
Animal Care and Management
The Mus musculus colonies employed in the present study were raised at the animal housing facility of the Institute of Zoology, University of the Punjab, Lahore, Pakistan, as described previously16. The mice were housed in stainless steel cages, and standard growth conditions were maintained, including a light-dark cycle (12 hours) and temperature (24 – 25 °C). They were provided with regular mouse chow containing 20% protein and had access to water ad libitum. A seven-day acclimatization period was allowed for the mice before the commencement of the experiment. Forty (40) healthy male albino mice (BALB/c), aged between 6 to 8 weeks, were chosen for the experimental trial. Subsequently, they were randomly assigned to four groups (n = 10): the control group (NC), PRP treated group (PC), alloxan treated diabetic group (DC), and alloxan + PRP treated group (DT). The random group allocation was performed after a one-week acclimatization period. Ethical approval for this study was granted by the Ethics Committee of the University of the Punjab, Lahore, Pakistan, under the reference number Ref/D/229/FIMS.
Preparation of PRP
A double centrifugation method was adopted as a standard protocol to prepare PRP10. EDTA-coated vacutainers filled with fresh human blood were centrifuged at 1600 revolutions per minute (rpm) for 10 minutes. An upper layer of plasma, a middle buffy coat, and an inner layer of erythrocytes were formed in the tubes. The plasma and upper half of the buffy coat were pipetted out into an Eppendorf tube. The second centrifugation at 2000 rpm for 10 minutes divided the plasma into two layers. The top platelet-poor plasma (PPP) was discarded, followed by a mild shake for the synthesis of homogenized platelet-rich plasma (Figure 1). The optimal number of platelets required for a regenerative stimulus lacks an absolute definition, but approximately 1,000,000 platelets/mL were used in the current study.
Animal Dosing, Sacrificing, and Sample Collection
The four groups (n = 10) were treated as follows:
After a 4-week period of PRP treatment, the mice were left fasted for 24 hours, and blood was collected through retro-orbital puncture as described previously16. Subsequently, the samples were subjected to centrifugation at 4,000 revolutions per minute (rpm) for 15 minutes at 4°C to isolate the serum and stored at -80°C until further analysis17. Following this, the mice were humanely euthanized using cervical dislocation. Liver tissues were meticulously excised, their weights recorded, and then divided into two distinct samples. One of these samples was promptly frozen at -80°C for subsequent analysis, while the other was preserved in a 10% formaldehyde solution for histopathological examination.
Biochemical Analysis
The serum insulin was assayed using an ELISA kit (Catalog Number RAB1317, Merck, Germany) according to the manufacturer’s instructions. For hepatic glycogen contents, the frozen liver sample was assayed using a biochemical kit (Catalog Number MET-5022, Cell Biolabs, USA).
Histological Procedure
To visualize glycogen deposition in the liver, we employed Periodic Acid-Schiff (PAS) staining. Formalin-fixed liver samples underwent a gradual dehydration process before being paraffin-embedded. Subsequently, these liver specimens were sectioned into 4 µm slices and subjected to PAS staining. Photomicrographs of the stained slides were captured using a light microscope equipped with a portable 'Ease-i-Imageur universal' camera.
RNA Extraction, Reverse Transcription, and Real-Time PCR
Total RNA extraction was performed using TRIzol reagent (Invitrogen, USA) followed by its quantification by a NanoDrop instrument (ND-1000, Thermo Scientific, USA) as previously reported17. Afterward, cDNA was synthesized using a ready-to-use kit (RevertAid First Strand cDNA Synthesis Kit, Cat #K1621, Thermo Scientific). The mRNA expression of selected genes was quantified using Maxima SYBR Green/ROX qPCR Master Mix (K0221, Thermo Scientific). Previously reported primers were used after their sequences were verified against the NCBI Blast database. The sequences were as follows: (GAPDH) Forward 5-GAAACCTGCCAAGTATGA-3; Reverse 5-GCTGTAGCCGTATTCATT-3, (Gp) Forward 5-AGTGAAAATCAACCCAGCCT-3; Reverse 5-CAGCGATGTTCTTGATCACC-3, (Gs) Forward 5-CCGGCTTTGGCTGCTTTAT-3; Reverse 5-CCGATCCAGAATGTAAATGCC-3, (Glut2) Forward 5-TTTGCAGTAGGCGGAATGG-3; Reverse 5-GCCAACATGGCTTTGATCCTT-3, (Glut4) Forward 5-CAACTGGACCTGTAACTTCATCGT-3; Reverse 5-ACGGCAAATAGAAGGAAGACGTA-3. To assess the relative expression of a specific gene, the 2ΔΔCt method was employed15.
Statistical Analysis
Prism GraphPad 9.1.0 (221) software was used to assess the data. A two-way ANOVA (analysis of variance) followed by Dunnett’s multiple comparisons test was used to identify statistically significant differences among groups CG to DT. P-values < 0.05 (*), < 0.01 (**), < 0.001 (***), or < 0.0001 (****).
Results
Serum insulin and glycogen content in hepatocytes
To assess whether the hypoglycemic effects of PRP were attributable to an enhancement in islet functionality, we quantified insulin levels within the serum. Our analysis revealed a statistically significant difference between the PRP-treated group (P = 0.0311) and the diabetic group (P = 0.0001). Furthermore, we investigated the influence of PRP on hepatic glycogen deposition by measuring its proportion in the liver. Notably, in the diabetic group, a significantly elevated level (P = 0.0012) was observed. Conversely, PRP treatment in the DT resulted in a substantial reduction (P = 0.0148) in hepatic glycogen levels (see Figure 3).
Histology
To confirm the presence of glycogen deposition within hepatocytes, liver sections were stained with PAS stain and subsequently observed under a microscope (Figure 4). Glycogen appeared as a distinct purple-red stain, while cell nuclei were stained light blue. In the hepatic sections from the control group, a typical and healthy liver microarchitecture was observed, with no detectable glycogen (Figure 4). Furthermore, the central vein, hepatic sinusoids, portal vein, and hepatocytes were all found to be structurally intact.
In contrast, the liver cells of the DC group exhibited a significant accumulation of glycogen within the cytoplasm, indicating pathological glycogen storage. However, treatment with PRP in the DT group markedly ameliorated glycogen accumulation within liver cells. PRP treatment effectively preserved the structural integrity of the hepatic lobule and maintained glycogen levels within the cells, comparable to those in the NC group. These findings suggest a therapeutic benefit of PRP in mitigating glycogen accumulation and preserving hepatic tissue structure (Figure 4).
Glycogen metabolism
To gain insights into the molecular mechanisms underlying glycogen metabolism and glucose transport, we selected four key genes: Gp, Gs, Glut2, and Glut4. Gp exhibited a statistically significant upregulation in the DC group (P = 0.0008), while it was significantly restored in the DT group (P = 0.0069). In contrast, the expression of Gs showed significant downregulation in DC (P = 0.0021) compared to the NC group (Figure 5).
The administration of PRP also had a notable impact on the expressions of Glut2 and Glut4. The DC group exhibited a significantly elevated expression of Glut2 (P = 0.0018) and Glut4 (P = 0.0049) compared to the NC group. Conversely, PRP treatment resulted in a significant upregulation of both Glut2 (P = 0.0175) and Glut4 (P = 0.0269), yielding promising outcomes. These findings suggest the potential hypoglycemic effects of PRP, which may lead to the inhibition of hepatic gluconeogenesis enzymes, subsequently reducing glucose output and enhancing glucose consumption and clearance.
Discussion
The therapeutic potential of growth factors (GFs) has been extensively investigated in the context of cell regeneration18. When administered in the form of platelet-rich plasma (PRP), these GFs have demonstrated the capacity to induce protective effects, modulate oxidative stress, and influence liver enzyme activity in alloxan-induced diabetic mice, as well as impact pancreatic microarchitecture19, 20. Additionally, PRP has shown promise in mitigating CCL4-induced liver injury21 and promoting the recovery of damaged testicular tissue22. Drawing from these prior investigations, we posit that the diverse bioactive components present in PRP collectively synergize to elicit anti-diabetic effects. These effects are characterized by improvements in insulin resistance, modulation of glycogen metabolism, and enhanced glucose uptake within hepatocytes.
In our study, we observed significant differences in serum insulin levels among PRP-treated DT and DC groups after four weeks of treatment. There was a decline in DC compared to NC, and it was restored in DT compared to DC after PRP treatment. A similar pattern has been consistently observed in prior investigations, suggesting that platelet-rich plasma (PRP) therapy may potentiate insulin secretion, possibly by enhancing pancreatic microarchitecture and rejuvenating beta cell functionality19, 23.
In histopathological examination, a conspicuous accumulation of glycogen was readily discernible within the tissues of the alloxan-induced diabetic group (DC). This finding aligns with the observations reported in a prior study24. In hepatocytes, there is excessive accumulation of glycogen, as illustrated in Figure 4. This phenomenon arises due to the insulin-independent influx of glucose into hepatocytes during episodes of hyperglycemia, subsequently followed by insulin-mediated conversion into hepatic glycogen4, 25. It is worth noting that elevated levels of both glucose and insulin are essential for this process to occur. Notably, our investigation demonstrates that PRP treatment results in a substantial reduction in glycogen content when comparing DT to DC.
To gain insights into the role of glycogen metabolism in DM, the expression of two key genes, Gp and Gs, was evaluated. Consistent with previous findings, upregulation was observed in Gp while the expression of Gs was downregulated in DC26. Dysregulation in glycogen metabolism may result in abnormal accumulation of glycogen in hepatic tissues observed in the current study (Figure 4). PRP treatment in diabetic mice dramatically increased glycogenolysis in the liver, as the expression of Gp was restored compared to the DC group. The mechanism behind the restoration of hepatic glycogen accumulation in DM is uncertain; it might be related to the ameliorating effect of GFs of PRP on pancreatic architecture23, followed by an increase in insulin secretion that modulates glycogen metabolism.
To demonstrate the role of glucose transporters in glucose homeostasis, the expression of Glut2 and Glut4 was assessed, and a significant decline was observed in alloxan-induced diabetic mice. PRP treatment in the DT group displayed promising restoration in their expression to near the standard value of the NC group. These outcomes highlighted the impact of PRP on manipulating Glut2 and Glut4 tasks, thus enhancing glucose uptake and improving diabetic conditions. This phenomenon may result in the suppression of the expression of pivotal gluconeogenic enzymes within the hepatic system, subsequently leading to a decrease in hepatic glucose production while simultaneously promoting increased glucose utilization and clearance27. PRP administration could assist glucose uptake in hepatocytes by encouraging the translocation of Glut2 and Glut4, which might partially cause the hypoglycemic potential of PRP in diabetic mice. Moreover, baseline platelet count, different dosages, and frequency of PRP administration can affect the potency of PRP therapy and could yield varying results28.
Conclusions
Current investigations have explored the physiological role of GFs in improving hepatic architecture and regulating glucose homeostasis via glycogen metabolism and glucose transport in diabetic mice. Although the exact mechanisms of these GFs and their therapeutic effects have not been identified, these effects are mainly attributed to their release from platelets and activation. However, this assumption needs further investigation.
Platelet-rich plasma is a cocktail of growth factors. However, the specific factors responsible for its therapeutic activity have not yet been identified. This incomplete knowledge of the exact composition and mode of action of PRP limits the ability to draw concrete conclusions about the efficacy of PRP therapy. Moreover, although the current study provides valuable insights from the mouse model, it does not address the absence of clinical data. Given the promising results in the current study and the consistent findings from prior investigations, we conclude the crucial role of growth factors in PRP and recommend the need for further investigation to explore the therapeutic potential of PRP in diabetes mellitus (DM).
Abbreviations
ANOVA - Analysis of Variance, BW - Body Weight, cDNA - Complementary DNA, DC - Diabetic Control group, DM - Diabetes Mellitus, DT - Diabetic mice treated with PRP, EDTA - Ethylenediaminetetraacetic Acid, ELISA - Enzyme-Linked Immunosorbent Assay, GAPDH - Glyceraldehyde 3-phosphate dehydrogenase (a reference gene), GFs - Growth Factors, Glut2 - Glucose Transporter type 2, Glut4 - Glucose Transporter type 4, IRS-1 - Insulin Receptor Substrate 1, NC - Normal Control, PAS - Periodic Acid-Schiff, PC - PRP Control group, PDGFs - Platelet-Derived Growth Factors, PF4 - Platelet Factor 4, PPP - Platelet-Poor Plasma, PRP - Platelet-rich plasma, qPCR - Quantitative Polymerase Chain Reaction, rpm - Revolutions Per Minute, S.E.M - Standard Error of the Mean, TGF-β - Transforming Growth Factor Beta
Acknowledgments
The authors are highly thankful to the Vice-Chancellor, University of the Punjab, Lahore, Pakistan for his support in accomplishing this research work.
Author’s contributions
AA & MS performed the major part of this research work, statistical analysis, interpretation of results, and initial draft. MBK revised the figures, improved the initial draft, and helped in lab work. AS & NK helped in lab work and preparation of initial draft. MHA and NS conceived the idea, supervised the work, revised, modified, and approved the final draft. All authors read and approved the final manuscript.
Funding
None.
Availability of data and materials
Data and materials used and/or analyzed during the current study are available from the corresponding author on reasonable request.
Ethics approval and consent to participate
Ethical approval for this study was granted by the Ethics Committee of the University of the Punjab, Lahore, Pakistan, under the reference number Ref/D/229/FIMS.
Consent for publication
Not applicable.
Competing interests
The authors declare that they have no competing interests.
References
-
Vieira
R.,
Souto
S.B.,
Sánchez-López
E.,
Machado
A.L.,
Severino
P.,
Jose
S.,
Sugar-lowering drugs for type 2 diabetes mellitus and metabolic syndrome-Review of classical and new compounds: Part-I. Pharmaceuticals (Basel, Switzerland).
2019;
12
(4)
:
152
.
View Article PubMed Google Scholar -
Lin
J.Y.,
Yin
R.X.,
Exposure to endocrine-disrupting chemicals and type 2 diabetes mellitus in later life. Exposure and Health.
2023;
15
(1)
:
199-229
.
View Article Google Scholar -
Colberg
S.R.,
Gillen
J.B.,
Francois
M.E.,
Type 2 diabetes, prediabetes, and gestational diabetes mellitus. Exercise to Prevent and Manage Chronic Disease Across the Lifespan.
2022;
2022
:
141
.
View Article Google Scholar -
Soon
G.S.,
Torbenson
M.,
The Liver and Glycogen: In Sickness and in Health. International Journal of Molecular Sciences.
2023;
24
(7)
:
6133
.
View Article PubMed Google Scholar -
Pérez-García
A.,
Hurtado-Carneiro
V.,
Herrero-De-Dios
C.,
Dongil
P.,
García-Mauriño
J.E.,
Sánchez
M.D.,
Storage and utilization of glycogen by mouse liver during adaptation to nutritional changes are GLP-1 and PASK dependent. Nutrients.
2021;
13
(8)
:
2552
.
View Article PubMed Google Scholar -
Żołnierkiewicz
O.,
Rogacka
D.,
Hyperglycemia - A culprit of podocyte pathology in the context of glycogen metabolism. Archives of Biochemistry and Biophysics.
2024;
753
:
109927
.
View Article PubMed Google Scholar -
Jiang
S.,
Young
J.L.,
Wang
K.,
Qian
Y.,
Cai
L.,
Diabetic- induced alterations in hepatic glucose and lipid metabolism: the role of type 1 and type 2 diabetes mellitus (Review). Molecular Medicine Reports.
2020;
22
(2)
:
603-11
.
View Article PubMed Google Scholar -
Gupta
S.,
Paliczak
A.,
Delgado
D.,
Evidence-based indications of platelet-rich plasma therapy. Expert Review of Hematology.
2021;
14
(1)
:
97-108
.
View Article PubMed Google Scholar -
Shen
S.,
Wang
F.,
Fernandez
A.,
Hu
W.,
Role of platelet-derived growth factor in type II diabetes mellitus and its complications. Diabetes & Vascular Disease Research ; Official Journal of the International Society of Diabetes and Vascular Disease.
2020;
17
(7)
:
1479164120942119
.
View Article PubMed Google Scholar -
Fahrner
A.,
Alchus Laiferová
N.,
Ukropcová
B.,
Ukropec
J.,
Krützfeldt
J.,
Activation of PDGF signaling in the adult muscle stem cell niche in patients with type 2 diabetes mellitus. The Journal of Clinical Endocrinology and Metabolism.
2023;
108
(8)
:
2052-64
.
View Article PubMed Google Scholar -
Guo
H.,
Wu
H.,
Li
Z.,
The pathogenesis of diabetes. International Journal of Molecular Sciences.
2023;
24
(8)
:
6978
.
View Article PubMed Google Scholar -
Tsutsumi
R.,
Ueberheide
B.,
Liang
F.X.,
Neel
B.G.,
Sakai
R.,
Saito
Y.,
Endocytic vesicles act as vehicles for glucose uptake in response to growth factor stimulation. Nature Communications.
2024;
15
(1)
:
2843
.
View Article PubMed Google Scholar -
Marushchak
M.,
Hevko
U.,
Krynytska
I.,
Insulin receptor substrate 1 gene variations and lipid profile characteristics in the type 2 diabetic patients with comorbid obesity and chronic pancreatitis. Endocrine Regulations.
2022;
56
(1)
:
1-9
.
View Article PubMed Google Scholar -
Verma
R.,
Kumar
S.,
Garg
P.,
Verma
Y.K.,
Platelet-rich plasma: a comparative and economical therapy for wound healing and tissue regeneration. Cell and Tissue Banking.
2023;
24
(2)
:
285-306
.
View Article PubMed Google Scholar -
Arif
A.,
Farooq
A.,
Abbasi
M.H.,
Khawar
M.B.,
Akhtar
T.,
Ali
H.A.,
Effect of heterologous platelet-rich plasma on liver and modulation of glucose metabolism and Wnt signalling pathways in diabetic mice. Molecular Biology Reports.
2023;
50
(9)
:
7145-54
.
View Article PubMed Google Scholar -
Khawar
M.B.,
Sheikh
N.,
Alterations in transaminase activity and serum level of leptin and hepcidin induced by high fat diet in albino rats. Journal of Biological Regulators and Homeostatic Agents.
2017;
31
(4)
:
951-6
.
PubMed Google Scholar -
Khawar
M.B.,
Liu
C.,
Gao
F.,
Gao
H.,
Liu
W.,
Han
T.,
Sirt1 regulates testosterone biosynthesis in Leydig cells via modulating autophagy. Protein & Cell.
2021;
12
(1)
:
67-75
.
View Article PubMed Google Scholar -
Mariani
E.,
Pulsatelli
L.,
Cattini
L.,
Dolzani
P.,
Assirelli
E.,
Cenacchi
A.,
Pure Platelet and Leukocyte-Platelet-Rich Plasma for Regenerative Medicine in Orthopedics-Time- and Preparation-Dependent Release of Growth Factors and Effects on Synovial Fibroblasts: A Comparative Analysis. International Journal of Molecular Sciences.
2023;
24
(2)
:
1512
.
View Article PubMed Google Scholar -
Nemati
M.,
Karbalaei
N.,
Mokarram
P.,
Dehghani
F.,
Effects of platelet-rich plasma on the pancreatic islet survival and function, islet transplantation outcome and pancreatic pdx1 and insulin gene expression in streptozotocin-induced diabetic rats. Growth Factors (Chur, Switzerland).
2020;
38
(3-4)
:
137-51
.
View Article PubMed Google Scholar -
Zarin
M.,
Karbalaei
N.,
Keshtgar
S.,
Nemati
M.,
Platelet-rich plasma improves impaired glucose hemostasis, disrupted insulin secretion, and pancreatic oxidative stress in streptozotocin-induced diabetic rat. Growth Factors (Chur, Switzerland).
2019;
37
(5-6)
:
226-37
.
View Article PubMed Google Scholar -
Abd Elzaher
F.,
Moussa
M.H.,
Raafat
M.H.,
Emara
M.M.,
Histological effect of platelet rich plasma on CCL4 induced liver fibrosis in adult albino rat. The Egyptian Journal of Histology.
2021;
44
:
932-40
.
-
Abdulla
A.K.,
Testicular Injection of Autologous Platelet-Rich Plasma (PRP) to Enhance the Sperm Parameters in Rabbit. Eurasian Medical Research Periodical.
2022;
7
:
171-9
.
-
Arif
A.,
Abbasi
M.H.,
Khawar
M.B.,
Sheikh
N.,
Effects of heterologous platelet-rich plasma on liver enzymes, oxidative stress biomarkers and pancreatic microarchitecture in diabetic mice. Kuwait Journal of Science.
2023;
50
(3)
:
300-6
.
View Article Google Scholar -
Lin
Y.,
Pan
J.,
Liu
Y.,
Yang
H.,
Wu
G.,
Pan
Y.,
Acanthopanax trifoliatus (L.) Merr polysaccharides ameliorates hyperglycemia by regulating hepatic glycogen metabolism in type 2 diabetic mice. Frontiers in Nutrition.
2023;
10
:
1111287
.
View Article PubMed Google Scholar -
Kumar
S.,
Senapati
S.,
Bhattacharya
N.,
Bhattacharya
A.,
Maurya
S.K.,
Husain
H.,
Mechanism and recent updates on insulin-related disorders. World Journal of Clinical Cases.
2023;
11
(25)
:
5840-56
.
View Article PubMed Google Scholar -
Zhang
W.Q.,
Zhao
T.T.,
Gui
D.K.,
Gao
C.L.,
Gu
J.L.,
Gan
W.J.,
Sodium butyrate improves liver glycogen metabolism in type 2 diabetes mellitus. Journal of Agricultural and Food Chemistry.
2019;
67
(27)
:
7694-705
.
View Article PubMed Google Scholar -
Liu
Y.,
Wang
C.,
Li
J.,
Mei
Y.,
Liang
Y.,
Hypoglycemic and hypolipidemic effects of phellinus linteus mycelial extract from solid-state culture in a rat model of type 2 diabetes. Nutrients.
2019;
11
(2)
:
296
.
View Article PubMed Google Scholar -
Shahbaz
A.,
Alzarooni
A.,
Veeranagari
V.R.,
Patel
K.,
Mohammed
C.,
Kuruba
V.,
Efficacy of Platelet-Rich Plasma Intra-articular Injections in Hip and Knee Osteoarthritis. Cureus.
2024;
16
(9)
:
e69656
.
View Article PubMed Google Scholar
Comments
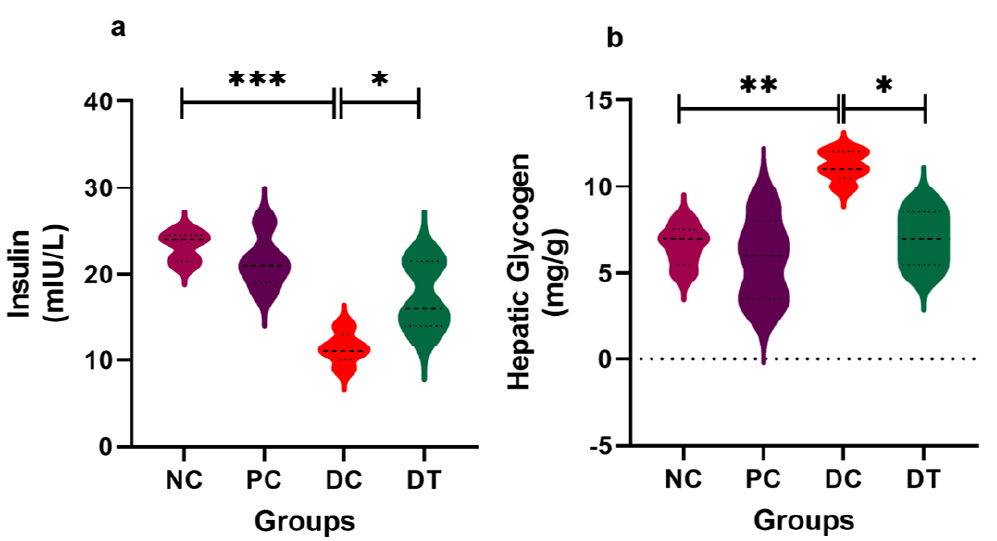
Article Details
Volume & Issue : Vol 11 No 11 (2024)
Page No.: 6932-6940
Published on: 2024-11-30
Citations
Copyrights & License

This work is licensed under a Creative Commons Attribution 4.0 International License.
Search Panel
Pubmed
Google Scholar
Pubmed
Google Scholar
Pubmed
Google Scholar
Pubmed
Google Scholar
Pubmed
Google Scholar
Pubmed
Google Scholar
Pubmed
Search for this article in:
Google Scholar
Researchgate
- HTML viewed - 585 times
- PDF downloaded - 195 times
- XML downloaded - 42 times