Abstract
Introduction: Orchophragmus violaceus (OV) has a rich history of use in traditional Chinese medicine, spanning millennia. Its diverse physiological properties, such as anti-radiation, antibacterial, antitumor, and hepatoprotective effects, have garnered widespread recognition. This study aims to elucidate the potential of OV extracts to mitigate radiation-induced intestinal injury (RIII) and its impact on the intestinal microbiota.
Method: OVS-2, extracted and isolated from OV seeds, was administered to C57 mice following abdominal irradiation (IR) with 60Co rays for RIII. Tissue samples from the small intestine and fecal matter were collected from three groups of mice three days post-administration for histological examination (HE staining) and 16S rRNA fecal intestinal flora sequencing. The composition of the intestinal flora was analyzed through 16S rRNA gene amplification.
Result: In vivo experiments demonstrated that OVS-2 supplementation improved the survival rate of mice and mitigated radiation-induced damage to intestinal villi and crypts to some extent. The relative abundance of Firmicutes, Bacillus, and Lactobacillus decreased in the experimental group compared to the model group, while that of Actinobacteria, Erysipelotrichaceae, and Dunaliella increased. LEfSe analysis revealed an increased relative abundance of Faecalibacterium, Dunaliella, Erysipelotrichales, and other bacteria, indicating their potential importance in the mouse intestinal microbiota. Dysbiosis was evident in the intestinal flora of mice in the IR group.
Conclusion: Oral administration of OVS-2 can help re-establish the gut microbiota composition and slow down the development of RIII in mice, suggesting that OVS-2 can regulate RIII and disturbance of intestinal flora in mice.
Introduction
Radiation-induced intestinal injury (RIII) is a severe complication of radiotherapy for abdominopelvic and retroperitoneal tumors, frequently occurring during the treatment of malignant abdominal and pelvic tumors1, 2, 3, 4. Patients often experience symptoms such as vomiting, weight loss, loss of appetite, diarrhea, and infection after radiotherapy5, 6, 7. In severe cases, the injury can lead to death from septic shock8. The mechanisms underlying radiation enteritis involve complex processes, including epithelial cell death, crypt stem cell damage, mucosal barrier dysfunction, and inflammation. Traditional interventions, like bone marrow transplantation, often fail to effectively resolve these issues9, 10, 11, 12, 13, 14, 15, 16.
Traditional Chinese medicine offers an effective treatment for RIII through its radioprotective, antioxidant, and anti-inflammatory properties17, 18. Orychophragmus violaceus, commonly known as the February orchid14, a natural antioxidant herb from the Brassicaceae family, has been extensively studied for its medicinal uses19, 20, including anti-radiation, antibacterial, antitumor, and hepatoprotective effects17, 18, 19, 20. The compounds in O. violaceus seeds mainly include alkaloids, flavonoids, and triterpenoid saponins, which possess physiological activities such as anti-free radical, antibacterial, antitumor, and hepatoprotective properties21. The intestinal flora, the largest microecological system in the human body, plays a crucial role in digestion and pathogen resistance22, 23, 24, 25. A disruption of the intestinal flora is a pivotal factor in RIII9, 26, 27, 28. Various studies have shown that traditional Chinese medicine can treat RIII by improving the intestinal flora29, 30, 31. This study aims to evaluate the therapeutic effects of OVS-2, an alkaloid extract from O. violaceus seeds, on RIII. We focus on its ability to enhance survival and alleviate gastrointestinal damage by modulating the gut microbiome, assessed through 16s rRNA fecal sequencing. This study aims to assess the impact of OVS-2 on the survival rates of mice subjected to abdominal radiation and analyze the changes in intestinal pathology and flora composition in response to OVS-2 treatment.
Methods
Preparation and Chemical Profiling of OVS-2
The herb, sourced from Shuyang (Anhui Province, China), was identified as Orychophragmus violaceus seeds of the genus Orychophragmus within the Cruciferae family. It was verified by Associate Researcher Li Bin from the Institute of Radiation Medicine, Academy of Military Medical Sciences. Sample specimens were securely stored in the laboratory.
The seeds were air-dried and extracted three times with 70% ethanol, each extraction lasting 2 hours. The concentrated extract was then processed by D001 cation-exchange resin with impurities removed through elution with water at a flow rate of 1 column volume per hour (BV/h). This was followed by elution with 95% ethanol containing 0.5% ammonia at a flow rate of 1 bpm. This process yielded the final product, OVS-2.
Animal Grouping
C57BL/6 mice were procured from SPF Biotechnology Co., Ltd. (Beijing, China) and acclimatized under standardized laboratory conditions for 7 days, with controlled temperature, humidity, and a 12/12-hour light/dark cycle. This study adhered to ethical guidelines approved by the Experimental Animal Medical Ethics Committee of the Military Medical Research Institute and was conducted in accordance with the principles of the 3Rs (Replacement, Reduction, Refinement). Mice were randomly assigned to one of three groups: a normal control group (CON), an irradiation group (IR), and a drug administration group (OVS-2). Randomization was ensured by using a computer-generated list. The study involved double-blinding, where the investigators administering the treatments and those assessing the outcomes were unaware of the group assignments. The weights of the mice were recorded before the start of the experiment. A 60Co irradiator from the Beijing Institute of Radiation Medicine was used to deliver a targeted abdominal irradiation dose of 15 Gy at a rate of 74.22 cGy/min, while non-abdominal areas were shielded with lead blocks. The ethical approval for this protocol was granted by the Animal Laboratory of the Experimental Animal Center, Military Medical Research Institute (Ethic number IACUC-DWZX-2023-P502, Approval date: 29 January 2022). Prior to irradiation, mice were anesthetized with an intraperitoneal injection of sodium pentobarbital to minimize distress. The control group received intragastric administration of normal saline, while the IR and OVS-2 groups received their respective treatments concurrently with irradiation. Specifically, the OVS-2 group was administered 100 mg/kg of OVS-2 orally 24 hours before irradiation, and subsequently at 0.5, 24, and 48 hours post-irradiation. The IR group received an equivalent volume of physiological saline following the same schedule.
Survival and Weight
All groups were monitored for survival and body weight changes over 30 days following a 15 Gy whole-abdominal irradiation.
HE Staining
On the 3.5th day post-irradiation, mice were euthanized by cervical dislocation. Small intestinal tissues were collected, fixed in 4% paraformaldehyde, stained with hematoxylin and eosin (HE), and sliced. The structure of the small intestinal mucosa was observed under a light microscope, and villus height and crypt depth were measured using ImageJ 3.
Intestinal Flora Sequencing
Genomic DNA was extracted from samples using an OMEGA DNA Kit (D5625-01) (Omega Bio-Tek, Norcross, GA, USA). The V3-V4 variable regions were PCR-amplified using specific primers and high-fidelity DNA polymerase, and the PCR products were detected by agarose gel electrophoresis. Target fragments were recorded and quantified using the Quant-iT PicoGreen dsDNA Assay Kit. The PCR amplification products were quantified using a microplate reader, and the ratio was adjusted according to the sequencing requirements. Libraries were constructed using the TruSeq Nano DNA LT library preparation kit, checked using Agilent Bioanalyzer 2100 and Promega QuantiFluor, and sequenced after qualification.
Statistical Analysis
Statistical analyses were performed using ImageJ and GraphPad Prism version 9.0.1. Survival analysis was performed using the Kaplan-Meier (K-M) curve, and the Log-rank test was used to compare survival rates between groups. Data are reported as mean ± standard deviation (SD). Differences between groups were analyzed using a two-way analysis of variance (ANOVA) and Tukey's analysis of variance to compare more than two groups. Alpha diversity refers to within-group diversity and was analyzed using the Tukey test. LEfSe analysis employs Linear Discriminant Analysis (LDA) and considers species with an LDA score greater than 4 as biomarkers with statistically significant differences between groups. STAMP difference analysis utilizes the Wilcoxon Rank-Sum Test to identify species with significant differences. Statistical significance was set at P < 0.05.
Results
Therapeutic Effect of OVS-2 on RIII in Mice
C57BL/6J mice were irradiated with 15 Gy 60Coγ-rays in the abdomen. OVS-2 was administered orally at a dose of 100 mg/kg 24 hours before irradiation, with subsequent administrations half an hour before irradiation, and 24 and 48 hours post-irradiation, while maintaining the same dosage as the initial oral administration, as shown in Figure 1A. Survival analysis using the Kaplan-Meier method and Log-rank test revealed that 100% of the mice in the OVS-2 group survived, compared to only 40% in the irradiated group without treatment (χ²=9.240, P < 0.05) (Figure 1B). H&E staining of intestinal tissues showed that the control mice had normal small intestinal villi, while the irradiated group showed disorganization, structural damage, and mild inflammation. In contrast, the OVS-2 treated mice had well-preserved intestinal structures, without significant damage or inflammation. Measurements confirmed that OVS-2 treatment resulted in longer villi and deeper crypts, suggesting that it effectively minimized radiation damage to the intestines (Figure 1C). These results showed that OVS-2 had strong anti-radiation activity in vivo.
Microbial Diversity Analysis
Alpha and Beta Diversity
Alpha diversity analysis reflects the abundance and diversity of the intestinal flora. These include the ACE, Chao 1, Shannon, and Simpson indices. Among them, the ACE and Chao 1 indices were used to estimate community richness, and the Shannon and Simpson indices were both used to estimate community diversity. The larger the two indices, the higher the community diversity. There was no statistically significant difference in the ACE and Chao 1 indices between the control and medication groups (P > 0.05) (Figure 2A and B). The Shannon and Simpson indices in the model group were both lower than those in the control and medication groups (P < 0.05), suggesting that species diversity decreased after irradiation and that OVS-2 increased species diversity post-irradiation (Figure 2C and D). Principal Coordinates Analysis (PCoA): PCoA analyses changes in the structure of the intestinal microbial community, used to study the similarity or difference in sample community composition. In this analysis, the proximity between points on the plot indicates more similar microbial compositions, whereas points that are farther apart represent greater differences. After radiation exposure, the structure of the intestinal bacteria changed greatly: the IR group was further away from the other two groups on the PCoA plot. This indicates a significant shift in microbial diversity due to radiation. In contrast, the distance between the CON group and the OVS-2 group was relatively close, suggesting that OVS-2 treatment helps maintain a microbial composition closer to that of the unirradiated state (Figure 2E).
Microbial Community Analysis
Phylum to Genus Level Distribution
At the phylum level, Firmicutes, Actinobacteria, and Proteobacteria dominated all three groups. The CON and OVS-2 groups exhibited the highest abundance of Actinobacteria, as shown in Figure 3A. At the class level, Actinobacteria, Bacteroidia, Bacilli, and Gammaproteobacteria dominated the three groups. The IR group had a higher abundance of Bacilli, whereas the CON and OVS-2 groups displayed a higher abundance of Actinobacteria. Additionally, the OVS-2 group exhibited a higher abundance of Clostridia compared to the other two groups. Varying degrees of changes were observed, as shown in Figure 3B. At the level of order classification of intestinal flora, the three groups are classified into Lactobacillaceae, Erysipelotrichales, Bifidobacteriales, Xanthomonadales, and Alteromonadales (with Burkholderiales being dominant). The abundance of Erysipelotrichales in the model group was significantly lower than that in the other two groups, and the abundance of Lactobacilli species was significantly higher than that in the other two groups. There were varying degrees of change among the species in each group, as shown in Figure 3C. At the family level, intestinal flora was classified into Streptococcaceae, Erysipelotrichaceae, Bifidobacteriaceae, Xanthomonadaceae, and Shewanellaceae. The abundance of Erysipelotrichaceae in the model group was significantly lower than that in the other two groups, and the abundance of Lactobacillus species was significantly higher than that in the other two groups. There were varying degrees of change among the species in each group, as shown in Figure 3D. At the genus level, the three groups were dominated by Streptococcus, Dubosiella, Allobaculum, Bifidobacterium, and Stenotrophomonas species. The abundance of Lactobacillus in the CON group was significantly higher than that in the other three groups, and there were varying degrees of changes among the species in each group, as shown in Figure 3E.
LEfSe Analysis for Biomarker Discovery
LEfSe analysis identifies factors and biomarkers with significant differences between categories. Through LEfSe analysis, we confirmed the presence of microorganisms with significant differences between groups (LDA > 4). Among them, bacteria that were significantly enriched in the CON group were f_Erysipelotrichaceae, o_Erysipelotrichales, g_Allobaculum, p_Actinobacteriota, o_Bifidobacteriales, c_Actinobacteria, f_Bifidobacteriaceae, g_Bifidobacterium, g_Dubosiella, and g_Faecalibaculum. The bacteria significantly enriched in the IR group were o_Lactobacillales, f_Streptococcaceae, g_Streptococcus, g_Lactococcus; f_Pasteurellaceae, o_Pasteurellales, and g_Rodentibacter. Bacteria significantly enriched in the OVS-2 group were o_Erysipelotrichales, f_Erysipelotrichaceae, g_Dubosiella, g_Faecalibaculum, p_Actinobacteriota, c_Clostridia, c_Actinobacteria, g_Bifidobacterium, f_Bifidobacteriaceae, and o_Bifidobacteriales (Figure 4A and B).
Significance Testing of Microbial Abundance
Significant Difference Testing compares the abundance of microbes in different groups using statistical tests to find any significant differences. This helps us understand which species are more abundant in one group compared to another. We analyzed the significant differences between the groups and found that the abundance of Streptococcus was higher in the IR group than that in the CON group. After drug intervention in the OVS-2 group, the abundance of these bacteria decreased significantly (P < 0.05), whereas the abundance of Dubosiella, Bifidobacterium, and Faecalibaculum in the IR group was low, but there was significant enrichment in both the CON and OVS-2 groups (P < 0.05) (Figure 4C and D).
Discussion
Our analysis highlighted no significant differences in alpha diversity (species richness) between the control (CON) and irradiated (IR) groups, while species diversity was lower in the IR group compared to both the CON and OVS-2 treated groups. This suggests that while radiation may not affect the richness of species, it does impact their distribution and abundance. The beta diversity results further support this, indicating a distinct separation in the microbial communities of the IR group compared to the CON and OVS-2 groups, with the latter showing a microbial composition more similar to the control.
Through Lefse analysis and significant difference test analysis between groups, we screened out the most advantageous beneficial bacteria of the OVS-2 group; beneficial bacteria that were significantly enriched in the OVS-2 group mainly included Dubosiella, Faecalibaculum, Bifidobacterium, Turicibacter, and Romboutsia.
The finding that OVS-2 treatment maintains closer microbial diversity to the control group aligns with studies highlighting the role of gut microbiota in radiation resistance. For instance, research has shown that microbiota diversity is crucial for maintaining gut homeostasis and resilience against external stressors such as radiation. The beneficial bacteria identified, including Dubosiella, Faecalibaculum, and Bifidobacterium, are known for their protective roles in gut health, which correlates with their observed enrichment in the OVS-2 group. Specifically, Faecalibacterium is renowned for its anti-inflammatory properties and has been shown to mitigate radiation-induced damage by enhancing mucosal integrity and reducing inflammation.
The therapeutic benefits of OVS-2 could be partly attributed to its modulation of butyric acid producers like Faecalibacterium and Dubosiella. Butyric acid is a critical short-chain fatty acid that maintains gut barrier function and regulates inflammatory responses. Therefore, the preservation of these bacteria could be crucial in mitigating the epithelial damage typically caused by radiation32, 33. Studies have shown that Faecalibacterium can reduce radiation-induced histological damage to the colon epithelium, prevent radiation-induced destruction of the colon epithelial barrier function, protect crypt epithelial progenitor/stem cell pools, differentiate epithelial tuft cells from colorectal irradiation, and maintain exposure, contributing to the self-renewal of irradiated colonic epithelium and reducing mucosal ulceration34, 35. Additionally, Bifidobacteria of the phylum Actinobacteria are common probiotics in the human intestine that play an important role in human health36, 37, 38. Bifidobacteria can acidify the intestinal environment, inhibit the growth of putrefactive and pathogenic bacteria, produce vitamins and amino acids to provide essential nutrients to the human body, stimulate immune responses, and reduce the occurrence of colon cancer39, 40, 41, 42, 43. This functionality is crucial for preventing the translocation of harmful pathogens and maintaining intestinal homeostasis. In a study on the treatment of patients with Crohn's disease (CD), Lindfors et al. found that bifidobacteria can upregulate the expression of ZO-1 and repair tight junctions between colonic epithelial cells44, suggesting that OVS-2 may protect the intestinal mucosal mechanical barrier through bifidobacteria.
Additionally, the Turicibacter bacterial group plays an important role in regulating host bile acid and lipid metabolism. Turicibacter strains alter host bile acid profiles, thereby reducing serum cholesterol, triglyceride levels, and adipose tissue mass. Colonizing strains of a single Turicibacter species induce changes in the host bile acid profile that are often consistent with those produced in vitro. Furthermore, colonizing mice with another bacterium that exogenously expresses a bile-modifying gene from a Turicibacter strain reduced serum cholesterol and triglyceride levels and the adipose tissue mass. These studies demonstrated that Turicibacter strains are capable of altering host bile acid and lipid metabolism genes and positioned Turicibacter bacteria as modulators of host lipid biology45, 46. Romboutsia is often associated with the patient's health status47, 48, and the dramatic decrease in Romboutsia abundance (-86.51%) in polyp-associated mucosa may represent a potential microbial indicator of the disease condition, i.e., Romboutsia may play a key role in health status and is a potential biomarker of intestinal dysbiosis49.
These results lay the groundwork for future explorations into the mechanisms by which OVS-2 modulates gut microbiota to confer radiation protection. Multi-omics approaches could provide deeper insights into the systemic effects of OVS-2, potentially uncovering novel therapeutic targets for managing radiation-induced intestinal injury (RIII). Continued research could also explore the scalability of OVS-2 treatment in clinical settings, assessing its efficacy and safety in larger populations.
Overall, our study not only confirms the protective effects of OVS-2 against radiation-induced intestinal damage but also provides a compelling argument for the therapeutic modulation of the gut microbiome as a viable strategy for enhancing radiation tolerance. Further investigations into the specific interactions between OVS-2 and intestinal flora will be crucial in developing refined interventions aimed at mitigating radiation's deleterious effects.
Conclusions
Our study confirms that OVS-2 enhances survival and reduces weight loss in mice after radiation, maintaining the integrity of intestinal structures and rebalancing gut flora. These results highlight OVS-2's effectiveness in both protecting the intestines and modulating the microbiome, making it a promising treatment for radiation-induced intestinal injury (RIII). This supports OVS-2's potential to improve treatment strategies for radiation damage, with further research needed to fully understand its benefits and mechanisms.
Abbreviations
16S rRNA - 16S ribosomal RNA, ACE - Abundance-based Coverage Estimator, ANOVA - Analysis of Variance, bpm - Beats per minute, BV/h - Bed Volume per hour, C57BL/6 - Mouse strain used in the study, CON - Control group, DNA - Deoxyribonucleic Acid, dsDNA - Double-stranded DNA, HE - Hematoxylin and Eosin, IACUC - Institutional Animal Care and Use Committee, IR - Irradiation, K-M - Kaplan-Meier, LDA - Linear Discriminant Analysis, LEfSe - Linear Discriminant Analysis Effect Size, OV - Orchophragmus violaceus, OVS-2 - Orychophragmus violaceus extract, PCR - Polymerase Chain Reaction, PCoA - Principal Coordinates Analysis, RIII - Radiation-Induced Intestinal Injury, SD - Standard Deviation, SPF - Specific Pathogen Free
Acknowledgments
None.
Author’s contributions
Conceptualization and experimental design: Qinglin Zhang, Fengjun Xiao; data collection and analysis: Haixia Li, Li Du, YuXin Lu; supervision and result interpretation: Xiaochen Cheng, Li Du, YuXin Lu; manuscript draft: Haixia Li; formal analysis, review and editing: Li Du, Fengjun Xiao. All authors commented on the previous versions of the manuscript, and read and approved the final manuscript.
Funding
None.
Availability of data and materials
Data and materials used and/or analyzed during the current study are available from the corresponding author on reasonable request.
Ethics approval
The Animal Laboratory of Experimental Animal Center, Military Medical Research Institute approved all animal experiments (Ethic number IACUC-DWZX-2023-P502, Approval date: 29 January 2022).
Consent for publication
Not applicable.
Competing interests
The authors declare that they have no competing interests.
References
-
Chen
G.,
Han
Y.,
Zhang
H.,
Tu
W.,
Zhang
S.,
Radiotherapy-Induced Digestive Injury: Diagnosis, Treatment and Mechanisms. Frontiers in Oncology.
2021;
11
:
757973
.
View Article PubMed Google Scholar -
Lu
L.,
Li
W.,
Chen
L.,
Su
Q.,
Wang
Y.,
Guo
Z.,
Radiation-induced intestinal damage: latest molecular and clinical developments. Future Oncology (London, England).
2019;
15
(35)
:
4105-18
.
View Article PubMed Google Scholar -
Lu
H.,
Yan
H.,
Li
X.,
Xing
Y.,
Ye
Y.,
Jiang
S.,
Single-cell map of dynamic cellular microenvironment of radiation-induced intestinal injury. Communications Biology.
2023;
6
(1)
:
1248
.
View Article PubMed Google Scholar -
Lu
Q.,
Liang
Y.,
Tian
S.,
Jin
J.,
Zhao
Y.,
Fan
H.,
Radiation-Induced Intestinal Injury: Injury Mechanism and Potential Treatment Strategies. Toxics.
2023;
11
(12)
:
1011
.
View Article PubMed Google Scholar -
Eltahawy
N.A.,
Elsonbaty
S.M.,
Abunour
S.,
Zahran
W.E.,
Synergistic effect of aluminum and ionizing radiation upon ultrastructure, oxidative stress and apoptotic alterations in Paneth cells of rat intestine. Environmental Science and Pollution Research International.
2017;
24
(7)
:
6657-66
.
View Article PubMed Google Scholar -
Suman
S.,
Kumar
S.,
Moon
B.H.,
Fornace
A.J.,
Kallakury
B.V.,
Datta
K.,
Increased Transgenerational Intestinal Tumorigenesis in Offspring of Ionizing Radiation Exposed Parent APC1638N/+ Mice. Journal of Cancer.
2017;
8
(10)
:
1769-73
.
View Article PubMed Google Scholar -
Zhang
C.,
Chen
K.,
Wang
J.,
Zheng
Z.,
Luo
Y.,
Zhou
W.,
Protective Effects of Crocetin against Radiation-Induced Injury in Intestinal Epithelial Cells. BioMed Research International.
2020;
2020
:
2906053
.
View Article PubMed Google Scholar -
Hauer-Jensen
M.,
Denham
J.W.,
Andreyev
H.J.,
Radiation enteropathy - pathogenesis, treatment and prevention. Nature Reviews. Gastroenterology & Hepatology.
2014;
11
(8)
:
470-9
.
View Article PubMed Google Scholar -
Fan
J.,
Lin
B.,
Fan
M.,
Niu
T.,
Gao
F.,
Tan
B.,
Research progress on the mechanism of radiation enteritis. Frontiers in Oncology.
2022;
12
:
888962
.
View Article PubMed Google Scholar -
Song
S.,
Chen
D.,
Ma
T.,
Luo
Y.,
Yang
Z.,
Wang
D.,
Molecular mechanism of acute radiation enteritis revealed using proteomics and biological signaling network analysis in rats. Digestive Diseases and Sciences.
2014;
59
(11)
:
2704-13
.
View Article PubMed Google Scholar -
Gandle
C.,
Dhingra
S.,
Agarwal
S.,
Radiation-Induced Enteritis. Clinical Gastroenterology and Hepatology.
2020;
18
(3)
:
39-40
.
View Article PubMed Google Scholar -
Wang
C.C.,
Ning
H.X.,
Li
H.,
Liang
M.J.,
Li
M.Y.,
Wang
S.,
[Research progress in prevention and treatment of radiation-induced intestinal injury by traditional Chinese medicine active components]. Zhongguo Zhongyao Zazhi.
2023;
48
(14)
:
3743-52
.
PubMed Google Scholar -
Lu
Q.,
Liang
Y.,
Tian
S.,
Jin
J.,
Zhao
Y.,
Fan
H.,
Radiation-Induced Intestinal Injury: Injury Mechanism and Potential Treatment Strategies. Toxics.
2023;
11
(12)
:
1011
.
View Article PubMed Google Scholar -
Zhang
K.,
Yang
Y.,
Zhang
X.,
Zhang
L.,
Fu
Y.,
Guo
Z.,
The genome of Orychophragmus violaceus provides genomic insights into the evolution of Brassicaceae polyploidization and its distinct traits. Plant Communications.
2023;
4
(2)
:
100431
.
View Article PubMed Google Scholar -
Shi
N.,
Research Overview on Orychophragmus spp. Plant. Journal of Anhui Agricultural Sciences.
2009;
7
(14)
:
6435-6460
.
-
Liu
D.,
Li
J.,
Zhang
S.,
Wang
X.,
Xiang
W.,
Zhang
J.,
Leaf spot disease of Orychophragmus violaceus caused by Alternaria tenuissima in China. Plant Disease.
2021;
105
(9)
:
2718
.
View Article PubMed Google Scholar -
Zhang
G.J.,
Li
B.,
Cui
H.M.,
Chen
L.,
Tian
Y.,
Liu
S.J.,
Orychophragines A-C, Three Biologically Active Alkaloids from Orychophragmus violaceus. Organic Letters.
2018;
20
(3)
:
656-9
.
View Article PubMed Google Scholar -
Pang
M.,
Shan
Y.,
Wang
F.F.,
Yang
S.X.,
Li
L.Y.,
Cao
L.,
[Protective effect of water extracts of Orychophragmus violaceus seeds on TAA-induced acute liver injury in mice]. Zhongguo Zhongyao Zazhi.
2020;
45
(6)
:
1399-405
.
PubMed Google Scholar -
Xu
Z.X.,
Li
B.,
Tian
Y.,
Li
M.,
Dong
J.X.,
Zhang
G.J.,
Pentacyclic spermidine alkaloids with radioprotective and anti-inflammatory activities from Orychophragmus violaceus. Organic & Biomolecular Chemistry.
2021;
19
(45)
:
9844-8
.
View Article PubMed Google Scholar -
Luo
H.Z.,
Jiang
H.,
Sun
B.,
Wang
Z.N.,
Jia
A.Q.,
Sesquiterpenoids and furan derivatives from the Orychophragmus violaceus (L.) O.E. Schulz endophytic fungus Irpex lacteus OV38. Phytochemistry.
2022;
194
:
112996
.
View Article PubMed Google Scholar -
Zhu
N.,
Wu
H.,
Xu
Z.,
Liu
C.,
Tian
Y.,
Hu
M.,
New alkaloids with unusual spermidine moieties from the seeds of Orychophragmus violaceus and their cytoprotective properties. RSC Advances.
2017;
7
(66)
:
41495-8
.
View Article Google Scholar -
Tang
T.,
Chen
H.G.,
Zhao
C.,
Gong
X.J.,
Deng
Q.F.,
Zhou
X.,
[Research progress on intestinal microecology regulating mechanism and biological activities of polysaccharides]. Zhongguo Zhongyao Zazhi.
2021;
46
(21)
:
5585-92
.
PubMed Google Scholar -
Zhang
L.,
Zhang
Z.,
Xu
L.,
Zhang
X.,
Maintaining the Balance of Intestinal Flora through the Diet: Effective Prevention of Illness. Foods.
2021;
10
(10)
:
2312
.
View Article PubMed Google Scholar -
Biedermann
L.,
Rogler
G.,
The intestinal microbiota: its role in health and disease. European Journal of Pediatrics.
2015;
174
(2)
:
151-67
.
View Article PubMed Google Scholar -
Yang
L.,
Wang
Y.,
Zhang
Y.,
Li
W.,
Jiang
S.,
Qian
D.,
Gut microbiota: a new avenue to reveal pathological mechanisms of constipation. Applied Microbiology and Biotechnology.
2022;
106
(21)
:
6899-913
.
View Article PubMed Google Scholar -
Li
Y.,
Zhang
Y.,
Wei
K.,
He
J.,
Ding
N.,
Hua
J.,
Review: Effect of Gut Microbiota and Its Metabolite SCFAs on Radiation-Induced Intestinal Injury. Frontiers in Cellular and Infection Microbiology.
2021;
11
:
577236
.
View Article PubMed Google Scholar -
Jian
Y.,
Zhang
D.,
Liu
M.,
Wang
Y.,
Xu
Z.X.,
The Impact of Gut Microbiota on Radiation-Induced Enteritis. Frontiers in Cellular and Infection Microbiology.
2021;
11
:
586392
.
View Article PubMed Google Scholar -
Yu
Y.,
Lin
X.,
Feng
F.,
Wei
Y.,
Wei
S.,
Gong
Y.,
Gut microbiota and ionizing radiation-induced damage: is there a link?. Environmental Research.
2023;
229
:
115947
.
View Article PubMed Google Scholar -
Cai
S.,
Xie
L.W.,
Xu
J.Y.,
Zhou
H.,
Yang
C.,
Tang
L.F.,
(-)-Epigallocatechin-3-Gallate (EGCG) Modulates the Composition of the Gut Microbiota to Protect Against Radiation-Induced Intestinal Injury in Mice. Frontiers in Oncology.
2022;
12
:
848107
.
View Article PubMed Google Scholar -
Gu
J.,
Zhao
L.,
Chen
Y.Z.,
Guo
Y.X.,
Sun
Y.,
Guo
Q.,
Preventive effect of sanguinarine on intestinal injury in mice exposed to whole abdominal irradiation. Biomedicine and Pharmacotherapy.
2022;
146
:
112496
.
View Article PubMed Google Scholar -
Wang
M.,
Dong
Y.,
Wu
J.,
Li
H.,
Zhang
Y.,
Fan
S.,
Baicalein ameliorates ionizing radiation-induced injuries by rebalancing gut microbiota and inhibiting apoptosis. Life Sciences.
2020;
261
:
118463
.
View Article PubMed Google Scholar -
Zhang
H.,
Xu
J.,
Wu
Q.,
Fang
H.,
Shao
X.,
Ouyang
X.,
Gut Microbiota Mediates the Susceptibility of Mice to Sepsis-Associated Encephalopathy by Butyric Acid. Journal of Inflammation Research.
2022;
15
:
2103-19
.
View Article PubMed Google Scholar -
Singh
V.,
Lee
G.,
Son
H.,
Koh
H.,
Kim
E.S.,
Unno
T.,
Butyrate producers, The Sentinel of Gut\textquotedblright: their intestinal significance with and beyond butyrate, and prospective use as microbial therapeutics. Frontiers in Microbiology.
2023;
13
:
1103836
.
View Article PubMed Google Scholar -
Lapiere
A.,
Geiger
M.,
Robert
V.,
Demarquay
C.,
Auger
S.,
Chadi
S.,
Prophylactic Faecalibacterium prausnitzii treatment prevents the acute breakdown of colonic epithelial barrier in a preclinical model of pelvic radiation disease. Gut Microbes.
2020;
12
(1)
:
1-15
.
View Article PubMed Google Scholar -
Martín
R.,
Rios-Covian
D.,
Huillet
E.,
Auger
S.,
Khazaal
S.,
Bermúdez-Humarán
L.G.,
Faecalibacterium: a bacterial genus with promising human health applications. FEMS Microbiology Reviews.
2023;
47
(4)
:
fuad039
.
View Article PubMed Google Scholar -
Cani
P.D.,
Neyrinck
A.M.,
Fava
F.,
Knauf
C.,
Burcelin
R.G.,
Tuohy
K.M.,
Selective increases of bifidobacteria in gut microflora improve high-fat-diet-induced diabetes in mice through a mechanism associated with endotoxaemia. Diabetologia.
2007;
50
(11)
:
2374-83
.
View Article PubMed Google Scholar -
Lee
J.H.,
O'Sullivan
D.J.,
Genomic insights into bifidobacteria. Microbiology and Molecular Biology Reviews.
2010;
74
(3)
:
378-416
.
View Article PubMed Google Scholar -
Chen
J.,
Chen
X.,
Ho
C.L.,
Recent Development of Probiotic Bifidobacteria for Treating Human Diseases. Frontiers in Bioengineering and Biotechnology.
2021;
9
:
770248
.
View Article PubMed Google Scholar -
Dominguez-Bello
M.G.,
De Jesus-Laboy
K.M.,
Shen
N.,
Cox
L.M.,
Amir
A.,
Gonzalez
A.,
Partial restoration of the microbiota of cesarean-born infants via vaginal microbial transfer. Nature Medicine.
2016;
22
(3)
:
250-3
.
View Article PubMed Google Scholar -
O'Callaghan
A.,
van Sinderen
D.,
Bifidobacteria and Their Role as Members of the Human Gut Microbiota. Frontiers in Microbiology.
2016;
7
:
925
.
View Article PubMed Google Scholar -
Mazziotta
C.,
Tognon
M.,
Martini
F.,
Torreggiani
E.,
Rotondo
J.C.,
Probiotics Mechanism of Action on Immune Cells and Beneficial Effects on Human Health. Cells.
2023;
12
(1)
:
184
.
View Article PubMed Google Scholar -
Wang
H.,
Huang
X.,
Tan
H.,
Chen
X.,
Chen
C.,
Nie
S.,
Interaction between dietary fiber and bifidobacteria in promoting intestinal health. Food Chemistry.
2022;
393
:
133407
.
View Article PubMed Google Scholar -
Mei
Z.,
Yuan
J.,
Li
D.,
Biological activity of galacto-oligosaccharides: A review. Frontiers in Microbiology.
2022;
13
:
993052
.
View Article PubMed Google Scholar -
Lindfors
K.,
Blomqvist
T.,
Juuti-Uusitalo
K.,
Stenman
S.,
Venäläinen
J.,
Mäki
M.,
Live probiotic Bifidobacterium lactis bacteria inhibit the toxic effects induced by wheat gliadin in epithelial cell culture. Clinical and Experimental Immunology.
2008;
152
(3)
:
552-8
.
View Article PubMed Google Scholar -
Hoffman
J.M.,
Margolis
K.G.,
Building community in the gut: a role for mucosal serotonin. Nature Reviews. Gastroenterology & Hepatology.
2020;
17
(1)
:
6-8
.
View Article PubMed Google Scholar -
Lynch
J.B.,
Gonzalez
E.L.,
Choy
K.,
Faull
K.F.,
Jewell
T.,
Arellano
A.,
Gut microbiota Turicibacter strains differentially modify bile acids and host lipids. Nature Communications.
2023;
14
(1)
:
3669
.
View Article PubMed Google Scholar -
Milani
C.,
Ticinesi
A.,
Gerritsen
J.,
Nouvenne
A.,
Lugli
G.A.,
Mancabelli
L.,
Gut microbiota composition and Clostridium difficile infection in hospitalized elderly individuals: a metagenomic study. Scientific Reports.
2016;
6
(1)
:
25945
.
View Article PubMed Google Scholar -
Ricaboni
D.,
Mailhe
M.,
Khelaifia
S.,
Raoult
D.,
Million
M.,
Romboutsia timonensis, a new species isolated from human gut. New Microbes and New Infections.
2016;
12
:
6-7
.
View Article PubMed Google Scholar -
Mangifesta
M.,
Mancabelli
L.,
Milani
C.,
Gaiani
F.,
de'Angelis
N.,
de'Angelis
G.L.,
Mucosal microbiota of intestinal polyps reveals putative biomarkers of colorectal cancer. Scientific Reports.
2018;
8
(1)
:
13974
.
View Article PubMed Google Scholar
Comments
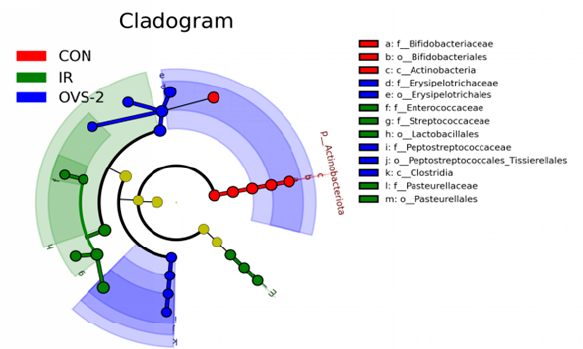
Article Details
Volume & Issue : Vol 11 No 9 (2024)
Page No.: 6764-6774
Published on: 2024-09-30
Citations
Copyrights & License

This work is licensed under a Creative Commons Attribution 4.0 International License.
Search Panel
Pubmed
Google Scholar
Pubmed
Google Scholar
Pubmed
Google Scholar
Pubmed
Google Scholar
Pubmed
Google Scholar
Pubmed
Search for this article in:
Google Scholar
Researchgate
- HTML viewed - 716 times
- PDF downloaded - 338 times
- XML downloaded - 37 times