Abstract
Introduction: Tamoxifen is a chemotherapy drug used in cancer treatment. In addition to its anticancer effect, tamoxifen also has hepatotoxic effects. This study aims to evaluate the induction effect of tamoxifen in the development of fatty liver in New Zealand white rabbits by employing ultrasonography.
Methods: Twenty female, adult New Zealand rabbits with a weight range of 1.5–2.5 kg and ages of 8–14 months were obtained from the Pasteur Institute in Tehran. After the adaptation period, the rabbits were randomly divided into four groups with 5 rabbits each. The first group, as a control group, received distilled water during the study period, and the other three groups received tamoxifen orally with daily doses of 1, 2, and 3 mg/kg body weight for 7 days. After 7 days of drug administration, a blood sample was taken from the middle ear artery of the rabbits to examine the serum levels of liver enzymes and assess several blood parameters. For liver imaging, a Mindray Z5 ultrasound machine with 5 MHz frequency and a linear probe was used to check the brightness of the liver before drug administration and 7 days after the final administration (i.e., day 14).
Results: The results show that tamoxifen dose-dependent dose-dependently caused a significant (p < 0.05) increase in alanine aminotransferase, aspartate aminotransferase, triglycerides, and low-density lipoprotein enzymes, and the amounts of high-density lipoprotein and alkaline phosphatase decreased significantly (p < 0.05). It should be noted that cholesterol changes were not significant (p > 0.05). From ultrasound imaging, tamoxifen changes the appearance of the liver from hyperechoic to hypoechoic.
Conclusions: The results show that oral administration of tamoxifen citrate at doses of 1, 2, and 3 mg/kg for 7 days causes liver damage and increases the leakage of liver enzymes and the levels of several blood parameters in New Zealand adult female rabbits, which can be evaluated by ultrasound imaging.
Introduction
Fatty liver is recognized as the most common cause of cirrhosis and liver failure, as well as one of the causes of liver cancer1. In addition, people with fatty liver are at increased risk of cardiovascular and cerebrovascular complications, hypertension, high blood lipids, and premature death2, 3, 4. Fatty liver disease also has more serious consequences in younger individuals and children. Thus, fatty liver disease should be considered a serious threat in national prevention and treatment programs5. Notably, laboratory methods are not sufficient for diagnosing fatty liver disease6. In most cases, fatty liver disease is asymptomatic and is accidentally discovered by observing elevated liver enzymes in blood tests performed for routine health checks or abdominal ultrasound performed for other reasons, although patients sometimes complain of vague upper right abdominal pain or early fatigue7.
The disease has two main causes, namely drugs or toxins and metabolic disorders. The metabolic syndrome involves a combination of high blood pressure, increased blood lipids, obesity, and diabetes, and recent studies indicate that as the number of diseases that make up this syndrome increases, the severity of fatty liver disease also increases8. Currently, it is believed that the progression of fatty liver disease toward cirrhosis is determined based on the intensity of chemical mediators produced during inflammation and the inflammatory process in liver cells; however, numerous chemical mediators may play a role in this pathway, but they have not been identified. Among them, leptin, angiotensin, and norepinephrine, which stimulate the proliferation of fibroblast-producing cells, require further investigation. These chemical mediators act unlike adiponectin, and by increasing fibrotic tissue, they cause rapid progression of the disease toward chronic and irreversible liver disease or cirrhosis9, 10. Clinical criteria, such as obesity, diabetes, hypertension, and being over 50 years old, are indicative of the severity of fatty liver disease. An increase in liver enzymes more than twice the normal limit and a level of triglycerides (TG) in the blood greater than 250 mg/dl are also laboratory criteria for the severity of fatty liver disease11.
Liver enzymes, such as aspartate aminotransferase and alanine aminotransferase, are present in liver cells and enter the serum upon destruction of the liver cells. These liver enzymes are the most strongly correlated with the increase in fatty liver disease12. Moreover, ultrasonography of the liver is the most common method for diagnosing fatty liver and is non-invasive, relatively inexpensive, and accessible, but does not have a high degree of correlation with histological changes. Therefore, imaging methods do not indicate the severity of fatty liver disease and cannot replace liver biopsy samples to determine the severity and prognosis of the disease13. Liver biopsy sampling is the most accurate method for evaluating the extent and severity of liver damage in individuals with a history or clinical suspicion of fatty liver disease14.
Tamoxifen is a non-steroidal anti-estrogen drug that has long been used to treat breast cancer, in addition to liver, brain, and pancreatic cancers15. However, it also has numerous side effects, including hot flashes and sweating, nausea and vomiting, loss of appetite, weight gain, vaginal discharge, irregular menstruation, excessive fatigue, headache, vaginal dryness, itching in the area surrounding the vagina, and more serious side effects such as liver disease, blood clots, depression, vision problems, and uterine or endometrial cancer16. Although the exact mechanism of action of the drug remains unclear, it likely works by blocking estrogen receptors in tumor cells that require estrogen for growth. The tamoxifen-estrogen receptor complex may enter the nucleus of tumor cells and inhibit DNA synthesis there17.
The present study focused on the evaluation of fatty liver disease induced by tamoxifen in New Zealand white rabbits, using ultrasound for the assessment. Fatty liver disease occurs because of the destruction of liver cells, and if not diagnosed early and properly treated, it can lead to an advanced and irreversible liver disease called cirrhosis. Fatty liver disease includes a spectrum of mild liver diseases in the form of fat accumulation in liver cells, which in its course may create inflammation of liver cells and result in the further destruction of liver cells. The coexistence of hypertension, increased blood fat, obesity, and diabetes, all of which are components of the metabolic syndrome18.
Results
To conduct this research, 20 female, adult New Zealand rabbits with a weight range of 1.5–2.5 kg and an age range of 8 to 14 months were obtained from the Pasteur Institute of Iran. Water and food were cut off for 6 hours before transporting the rabbits to the laboratory environment (animal house of the Faculty of Veterinary Medicine, University of Science and Research), and all rabbits were kept in specially designed transport cages and cared for following international ethical and health guidelines for animal welfare. To examine their health after transferring them to the laboratory, all animals were examined for physical health, and animals with suspicious symptoms were not included in the study. To allow the animals to adapt to the environment and become accustomed to the researcher, they were kept under 12-hour light-dark cycles, fed based on 5% of their body weight, provided free access to water, maintained at a temperature of approximately 22 °C and relative humidity of 55%, and prevented from undergoing any prior experiments or stress. In the next stage, the rabbits were randomly and equally divided into four groups of 5:
Group 1 (Control): Received placebo orally
Group 2 (Treatment): Received tamoxifen orally at a dose of 1 mg/kg for 7 days
Group 3 (Treatment): Received tamoxifen orally at a dose of 2 mg/kg for 7 days
Group 4 (Treatment): Received tamoxifen orally at a dose of 3 mg/kg for 7 days
Sampling was performed in two stages; once before the start of the experiment to determine the normal range, and again 7 days after the final daily administration of tamoxifen (i.e., on the 14th day). On the day of sampling, all animals underwent a 12-hour fast and only had access to water. The central artery of the ear was used to obtain blood samples. First, the hair in the desired area was shaved, and then the area was disinfected with alcohol and betadine. Next, using an insulin syringe and a suitable angiocatheter, 3 cc of fresh blood was collected into yellow-capped citrate tubes and transferred to the laboratory under standard conditions while maintaining the cold chain in less than an hour. Using a Prestige 24i AutoAnalyzer, the TG was measured based on GPO-PAP, aspartate aminotransferase (AST) and alanine aminotransferase (ALT) were measured based on IFCC without pyridoxal phosphate, and low-density lipoprotein (LDL) was measured by enzymatic determination.
To perform the ultrasound, the hair in the abdominal area of the animal was shaved using a modified Moser TiG40 shaver, and then the animal was placed on a special table in a supine position. Ultrasound was performed by an experienced radiologist using a Mindray Z5 machine and a linear probe before drug administration and 14 days after beginning administration, and the brightness level of the liver was evaluated. Based on the echogenicity of the liver and its comparison with adjacent kidneys, as well as the comparison of the spleen and the appearance of the diaphragm and the margin of intrahepatic vessels, the animals were classified into four groups: healthy, mild fatty liver (GI), moderate fatty liver (GII), and severe fatty liver (GIII). After performing the sonography and obtaining blood samples, the animals were removed from the study, for which a decapitator was used under anesthesia, and all ethical principles and animal rights were observed.
SPSS statistical software (version 21) was used for data analysis. The sample size was estimated to be 10 New Zealand rabbits based on the Cochran formula at a 0.05 level of error, and the Pearson correlation test, dependent t-test, and Kolmogorov–Smirnov test were used to determine the normality of the data distribution.
Variable | Time | Mean | Standard deviation |
---|---|---|---|
Alanine transaminase enzyme (international unit per liter) | 1 st day | 50 | 11.59 |
21 st day | 65 | 10.26 | |
Aspartate transaminase enzyme (international unit per liter) | 1 st day | 25.66 | 0.33 |
21 st day | 36.66 | 1.85 | |
Alkaline phosphatase enzyme (international unit per liter) | 1 st day | 293.67 | 17.57 |
21 st day | 230.33 | 38.8 | |
Blood cholesterol (mg/dL) | 1 st day | 126.33 | 40.10 |
21 st day | 149.33 | 58.26 | |
Blood triglycerides (mg/dL) | 1 st day | 61 | 5.13 |
21 st day | 98 | 15.04 | |
High density lipoprotein (mg/dL) | 1 st day | 77 | 22.23 |
21 st day | 31.33 | 6.22 | |
Low density lipoprotein (mg/dL) | 1 st day | 46.33 | 14.62 |
21 st day | 83 | 41.40 |
Variable | Time | Mean | Standard deviation |
---|---|---|---|
Alanine transaminase enzyme (international unit per liter) | 1 st day | 44 | 17 |
21 st day | 75.66 | 15.60 | |
Aspartate transaminase enzyme (international unit per liter) | 1 st day | 30.33 | 0.84 |
21 st day | 36.66 | 2.02 | |
Alkaline phosphatase enzyme (international unit per liter) | 1 st day | 314.67 | 28.22 |
21 st day | 174 | 56.29 | |
Blood cholesterol (mg/dL) | 1 st day | 110.33 | 24.25 |
21 st day | 135 | 35.11 | |
Blood triglycerides (mg/dL) | 1 st day | 48.66 | 3.28 |
21 st day | 172 | 99.59 | |
High density lipoprotein (mg/dL) | 1 st day | 70 | 12.16 |
21 st day | 31.33 | 5.66 | |
Low density lipoprotein (mg/dL) | 1 st day | 36.33 | 1.76 |
21 st day | 68.66 | 25.18 |
Variable | Time | Mean | Standard deviation |
---|---|---|---|
Alanine transaminase enzyme (international unit per liter) | 1 st day | 43.33 | 9.52 |
21 st day | 54 | 14.29 | |
Aspartate transaminase enzyme (international unit per liter) | 1 st day | 23.33 | 3.38 |
21 st day | 23 | 4.72 | |
Alkaline phosphatase enzyme (international unit per liter) | 1 st day | 277 | 40.67 |
21 st day | 161 | 51.59 | |
Blood cholesterol (mg/dL) | 1 st day | 115.66 | 13.77 |
21 st day | 192.33 | 72.39 | |
Blood triglycerides (mg/dL) | 1 st day | 38.66 | 7.35 |
21 st day | 62.66 | 8.41 | |
High density lipoprotein (mg/dL) | 1 st day | 69 | 14.18 |
21 st day | 36.33 | 2.84 | |
Low density lipoprotein (mg/dL) | 1 st day | 32.33 | 1.20 |
21 st day | 107.67 | 52.20 |
Variable | Sig. (2-tailed) | T-value |
---|---|---|
ALT1 - ALT21 | 0.004 | - 3.67 |
AST1 - AST21 | 0.005 | - 3.49 |
ALP1 - ALP21 | 0.005 | 3.51 |
Chol1 - Chol21 | 0.11 | - 1.70 |
TG1 - TG21 | 0.01 | - 2.78 |
HDL1 - HDL21 | < 0.001 | 7.11 |
LDL1 - LDL21 | 0.02 | - 2.59 |
Results
Ultrasound data analysis
The results of the ultrasound examination showed that changes in the liver progressed more quickly with an increase in the dose of tamoxifen, changing from hypoechoic to hyperechoic (Figure 1, Figure 2, Figure 3, Figure 4, Figure 5, Figure 6, Figure 7). For example, in the treatment group receiving 1 mg/kg of tamoxifen, these changes progressed toward increased echogenicity (hyperechogenicity), but in the treatment group receiving 2 mg/kg of tamoxifen, the changes progressed toward hypoechoic (loss of granularity), and in some cases, hyperechoic with complete loss of echo texture. In the group treatment receiving the maximum dosage, 3 mg/kg of tamoxifen, complete hyperechogenicity of the liver in the ultrasound examination, accompanied by cross-linking or softening in some rabbits.
Analysis of blood factors
The statistical values for the treatment groups with doses of 1 (Table 1), 2 (Table 2), and 3 mg/kg (Table 3) were obtained. The results of the t-test are also presented in Table 4. A significant increase in the alanine aminotransferase enzyme (p = 0.004) was observed in all treatment groups. Furthermore, no significant difference in this enzyme increase was observed among the treatment groups (p ≥ 0.05). Moreover, the t-test revealed a value of 67.3 for the alanine transaminase enzyme index.
A significant increase in the aspartate transaminase enzyme (p = 0.005) was observed in all treatment groups, and no significant difference in this enzyme increase was observed among the groups (p ≥ 0.05). The t-test revealed a value of 49.3 for the aspartate transaminase enzyme index.
A significant decrease was observed in the alkaline phosphatase (ALP) enzyme (p = 0.005) was observed in all treatment groups, and no significant difference in this enzyme decrease was observed among the groups (p ≥ 0.05). Meanwhile, the t-test revealed a value of 51.3 for the ALP enzyme index.
No significant changes were observed in the blood cholesterol levels (p = 0.11) for any of the groups, and no significant difference in the change in blood cholesterol levels was observed among the groups (p ≥ 0.05). At the same time, the t-test revealed a value of 70.1 for the cholesterol index.
A significant increase in blood triglyceride levels (p = 0.01) was observed in all treatment groups, and no significant difference in this increase was observed among the groups (p ≥ 0.05). The t-test revealed a value of 78.2 for blood TG.
A significant decrease in high-density lipoprotein (HDL) (p < 0.001) was observed in all treatment groups, and no significant difference in this decrease was observed among the groups (p ≥ 0.05). The t-test revealed a value of 11.7 for HDL.
A significant increase in LDL (p = 0.02) was observed in all treatment groups, and no significant difference in this increase was observed among the groups (p ≥ 0.05). Finally, the t-test revealed a value of 59.2 for LDL.
Examining the relationship between laboratory findings and ultrasound results
Comparing the laboratory data and ultrasound findings, there is a significant relationship between the increase in the serum levels of some enzymes and the brightness of the liver. Specifically, increasing the dose of tamoxifen from 1 to 3 mg/kg causes a significant increase (p ≤ 0.05) in the ALT, AST, TG, and LDL enzymes. The higher the amount of these factors in the blood, the more the appearance of the liver changes from hypoechoic to hyperechoic. For the oral dose of 3 mg/kg, multiple samples exhibit hyperechoic liver tissue, but in the 1 mg/kg group, the samples exhibit hypoechoic liver tissue. In other words, the changes in the blood factors are consistent with the changes in the brightness of the liver image, and the non-alcoholic fatty liver caused by tamoxifen consumption can be identified and investigated using diagnostic ultrasound.
Discussion
This study showed that the use of tamoxifen can increase the levels of certain liver and blood factors in a dose-dependent manner, accelerating the onset of fatty liver disease. Mitochondria are important organelles within a cell, responsible for various functions such as supplying adenosine triphosphate, regulating cellular acidity, and regulating osmotic pressure19. In the case of mitochondrial dysfunction, the energy needed to perform cellular activities is not produced, and cell death occurs owing to the disruption of intracellular organelles20. One of the main functions of tamoxifen in cells is to disrupt the cellular respiratory chain in mitochondria, by hindering the electron transfer process in the bioenergetic pathway of mitochondria, thus halting the energy production in the cell21. If this disruption occurs in liver cells, hepatocyte death and chronic or acute liver damage may occur22.
Khanam investigated the toxicity of cyclophosphamide methotrexate fluorouracil (CMF) chemotherapy in rabbits and found that rabbits who had received tamoxifen in addition to CMF had higher levels of AST and ALT enzymes compared with groups that did not receive tamoxifen23. In that study, the level of lactate dehydrogenase enzyme also increased in the tamoxifen group compared with the control group. Additionally, histopathological evaluations showed that the livers of mice receiving tamoxifen underwent pathological changes such as liver edema, portal vein congestion, and fibrosis. Those results are consistent with the present study, showing that different doses of tamoxifen caused liver damage and subsequent tissue changes in rabbits, and increased hepatic leakage enzymes. Although the exact mechanism of fatty liver disease caused by tamoxifen has not been identified, numerous studies suggest that the drug impairs mitochondrial function by reducing the activity of fatty acid oxidation in mitochondria. Specifically, tamoxifen may play a role in causing fatty liver disease by disrupting the function of carnitine palmitoyltransferase, which is one of the key enzymes involved in the fatty acid oxidation process in mitochondria. Cardosa et al. investigated the effects of tamoxifen on the respiratory rate and mitochondrial phosphorylation efficiency in rats, showing that tamoxifen reduces the production of adenosine triphosphate and even leads to cell death in liver cells24. They showed that tamoxifen and its metabolites directly and indirectly affect the bioenergetics of mitochondria and have detrimental effects on the liver in both the short and long term. Their results are consistent with the present work, demonstrating the harmful effects of different tamoxifen doses on liver cells. However, the efficacy of tamoxifen in treating breast cancer and improving tumor masses often overshadows its hepatic effects.
In a similar study focused on the hepatotoxic effects of tamoxifen in female rats, it was found that intraperitoneal injection of 45 mg/kg for 10 consecutive days increased the levels of liver enzymes such as ALT, AST, and ALP while decreasing the levels of some cellular oxidative enzymes, such as glutathione peroxidase, glutathione reductase, superoxide dismutase, and catalase25. Histological examinations of the liver also revealed that tamoxifen treatment induced pathological changes in the liver and increased the tumor necrosis factor levels. Other studies have shown that in addition to damaging cellular mitochondria, tamoxifen also plays a role in DNA damage. One of the main mechanisms of the drug’s anticancer effect is to affect cellular genetic material. This liver damage caused by active metabolites of tamoxifen leads to cell death in hepatocytes. The metabolism of tamoxifen in the liver and the release of toxic precursors contribute to the pathological changes in the liver. Male Swiss albino rats were used in a study on the hepatotoxic effect of tamoxifen in rats, and the levels of multiple blood and liver factors, including superoxide dismutase, glutathione peroxidase, glutathione reductase, dehydrogenase phosphate, cytochrome P450 content, and low molecular weight antioxidants, were measured26. The results showed that tamoxifen causes lipid peroxidation and inhibits antioxidant defense enzymes, leading to liver damage. In the present study, tamoxifen was found to increase the leakage of certain liver enzymes, as confirmed by sonographic imaging. Furthermore, with an increase in the dose, the liver changed from hyperechoic to hypoechoic, becoming softer. In another study focused on the hepatotoxic effect of tamoxifen and its impact on the pituitary glands in rats, it was found that intraperitoneal injection of 6 mg/kg of tamoxifen for 2 weeks caused changes in the serum levels of aspartate and aminotransferase enzymes27. Liver sampling performed on the last day of the study showed that some liver hepatocytes were swollen, and their nuclei were distributed unevenly and pycnotic. Most hepatocyte nuclei were also characterized by thick and distinct heterochromatin. In addition to these observations, electron microscopy studies indicated a disorder in the inner membranes of mitochondria, and the measurement of apoptotic factors revealed an increase in caspase-3 levels. The results of this study are consistent with the present study, indicating that liver damage is a direct consequence of tamoxifen use. Another reason for the occurrence of cellular damage following tamoxifen use is the increased amount of free electrons transferred to the mitochondrial membrane. With an increase in the level of these electrons, the chance of their connection to intracellular oxygen and the formation of reactive species, superoxides, and peroxides increases. In other words, the increase in free radicals due to the increase in wandering electrons in the respiratory chain is another mechanism of hepatic cellular damage resulting from tamoxifen use.
In the present study, it was found that the oral administration of 1, 2, and 3 mg/kg of tamoxifen for 7 days resulted in a significant increase in several blood and liver factors, including AST, ALT, TG, and LDL cholesterol, and a decrease in other factors, such as ALP and HDL cholesterol. Changes in the blood and liver parameters were confirmed by an increase in liver leakage enzymes and by sonographic imaging. Despite the hepatotoxic effects of tamoxifen, its popularity for the treatment of breast cancer will not be diminished. In various studies, hepatoprotective drugs have been used to control the hepatotoxic effect of tamoxifen. It may be possible to reduce the adverse effects of tamoxifen on the liver by increasing protective enzymes and improving the function of hepatocytes.
Conclusions
This study showed that tamoxifen citrate orally administered at doses of 1, 2, and 3 mg/kg for 7 days causes liver damage and increases the leakage of liver enzymes. These changes were not only determined based on the measurements of blood samples but also visualized by ultrasound imaging, with the liver appearance shifting from hyperechoic to hypoechoic.
Abbreviations
ALP: alkaline phosphatase, ALT: alanine transaminase, AST: aspartate transaminase, Chol: cholesterol, CMF: Cyclophosphamide Methotrexate Fluorouracil, HDL: high-density lipoprotein, LDL: low density lipoprotein, TG: triglyceride
Acknowledgments
None.
Author’s contributions
Conceptualization, MS-A; Formal analysis, MS-A and MNM; Funding acquisition, MS-A; Investigation, MS-A and VK; Methodology, MS-K and MNM; Supervision, MNM; Validation, VK; Writing – original draft, MNM; Writing – review & editing, MS-A, MNM and VK. All authors read and approved the final manuscript.
Funding
None.
Availability of data and materials
Data and materials used and/or analyzed during the current study are available from the corresponding author on reasonable request.
Ethics approval and consent to participate
The research design was approved by the ethical committee and institutional review board of Science and Research Branch, Islamic Azad University, Tehran (IR.IAU.SRB.REC.1401.197).
Consent for publication
Not applicable.
Competing interests
The authors declare that they have no competing interests.
References
-
Kanwal
F.,
Kramer
J.R.,
Duan
Z.,
Yu
X.,
White
D.,
El-Serag
H.B.,
Trends in the burden of nonalcoholic fatty liver disease in a United States cohort of veterans. Clinical Gastroenterology and Hepatology.
2016;
14
(2)
:
301-8
.
View Article Google Scholar -
Misra
V.L.,
Khashab
M.,
Chalasani
N.,
Nonalcoholic fatty liver disease and cardiovascular risk. Current Gastroenterology Reports.
2009;
11
(1)
:
50-5
.
View Article Google Scholar -
Oikonomou
D.,
Georgiopoulos
G.,
Katsi
V.,
Kourek
C.,
Tsioufis
C.,
Alexopoulou
A.,
Non-alcoholic fatty liver disease and hypertension: coprevalent or correlated?. European Journal of Gastroenterology & Hepatology.
2018;
30
(9)
:
979-85
.
View Article Google Scholar -
Chatrath
H.,
Vuppalanchi
R.,
Chalasani
N.,
Dyslipidemia in patients with nonalcoholic fatty liver disease. InSeminars in liver disease.
2012;
32
(0)
:
022-9
.
View Article Google Scholar -
Clark
J.M.,
Brancati
F.L.,
Diehl
A.M.,
Nonalcoholic fatty liver disease. Gastroenterology.
2002;
122
(6)
:
1649-57
.
View Article Google Scholar -
Pacifico
L.,
Celestre
M.,
Anania
C.,
Paolantonio
P.,
Chiesa
C.,
Laghi
A.,
MRI and ultrasound for hepatic fat quantification:relationships to clinical and metabolic characteristics of pediatric nonalcoholic fatty liver disease. Acta Paediatrica (Oslo, Norway).
2007;
96
(4)
:
542-7
.
View Article Google Scholar -
Newton
J.L.,
Systemic symptoms in non-alcoholic fatty liver disease. Digestive Diseases (Basel, Switzerland).
2010;
28
(1)
:
214-9
.
View Article Google Scholar -
Stefan
N.,
Häring
H.U.,
Cusi
K.,
Non-alcoholic fatty liver disease: causes, diagnosis, cardiometabolic consequences, and treatment strategies. The Lancet. Diabetes & Endocrinology.
2019;
7
(4)
:
313-24
.
View Article Google Scholar -
Brunt
E.M.,
Pathology of nonalcoholic fatty liver disease. Nature Reviews. Gastroenterology & Hepatology.
2010;
7
(4)
:
195-203
.
View Article Google Scholar -
Brunt
E.M.,
Wong
V.W.,
Nobili
V.,
Day
C.P.,
Sookoian
S.,
Maher
J.J.,
Nonalcoholic fatty liver disease. Nature Reviews. Disease Primers.
2015;
1
(1)
:
15080
.
View Article Google Scholar -
Sanyal
A.J.,
Past, present and future perspectives in nonalcoholic fatty liver disease. Nature Reviews. Gastroenterology & Hepatology.
2019;
16
(6)
:
377-86
.
View Article Google Scholar -
Festi
D.,
Schiumerini
R.,
Marzi
L.,
Biase
A.R. Di,
Mandolesi
D.,
Montrone
L.,
Review article: the diagnosis of non-alcoholic fatty liver disease \textemdash availability and accuracy of non-invasive methods. Alimentary Pharmacology & Therapeutics.
2013;
37
(4)
:
392-400
.
View Article Google Scholar -
Joy
D.,
Thava
V.R.,
Scott
B.B.,
Diagnosis of fatty liver disease: is biopsy necessary?. European Journal of Gastroenterology & Hepatology.
2003;
15
(5)
:
539-43
.
View Article Google Scholar -
Torres
D.M.,
Williams
C.D.,
Harrison
S.A.,
Features, diagnosis, and treatment of nonalcoholic fatty liver disease. Clinical Gastroenterology and Hepatology.
2012;
10
(8)
:
837-58
.
View Article Google Scholar -
Buckley
M.M.,
Goa
K.L.,
Tamoxifen. A reappraisal of its pharmacodynamic and pharmacokinetic properties, and therapeutic use. Drugs.
1989;
37
(4)
:
451-90
.
View Article Google Scholar -
Love
R.R.,
Tamoxifen therapy in primary breast cancer: biology, efficacy, and side effects. Journal of Clinical Oncology.
1989;
7
(6)
:
803-15
.
View Article Google Scholar -
Lam
H.Y.,
Tamoxifen is a calmodulin antagonist in the activation of cAMP phosphodiesterase. Biochemical and Biophysical Research Communications.
1984;
118
(1)
:
27-32
.
View Article Google Scholar -
Cohen
J.C.,
Horton
J.D.,
Hobbs
H.H.,
Human fatty liver disease: old questions and new insights. Science.
2011;
332
(6037)
:
1519-23
.
View Article Google Scholar -
Lodish
H.,
Berk
A.,
Kaiser
C.A.,
Kaiser
C.,
Krieger
M.,
Scott
M.P.,
Molecular cell biologyMacmillan 2008.
Google Scholar -
Chen
H.,
Chan
D.C.,
Mitochondrial dynamics fusion, fission, movement, and mitophagy in neurodegenerative diseases. Human Molecular Genetics.
2009;
18
:
169-76
.
View Article Google Scholar -
Larosche
I.,
Lettéron
P.,
Fromenty
B.,
Vadrot
N.,
Abbey-Toby
A.,
Feldmann
G.,
Tamoxifen inhibits topoisomerases, depletes mitochondrial DNA, and triggers steatosis in mouse liver. The Journal of Pharmacology and Experimental Therapeutics.
2007;
321
(2)
:
526-35
.
View Article Google Scholar -
Abid-Essefi
S.,
Ouanes
Z.,
Hassen
W.,
Baudrimont
I.,
Creppy
E.,
Bacha
H.,
Cytotoxicity, inhibition of DNA and protein syntheses and oxidative damage in cultured cells exposed to zearalenone. Toxicology In Vitro.
2004;
18
(4)
:
467-74
.
View Article Google Scholar -
Khanam
A.,
Toxicities induced by CMF chemotherapy in Rabbits. World Journal of Pharmaceutical Sciences..
2014;
2
(9)
:
981-7
.
-
Cardoso
C.M.,
Custódio
J.B.,
Almeida
L.M.,
Moreno
A.J.,
Mechanisms of the deleterious effects of tamoxifen on mitochondrial respiration rate and phosphorylation efficiency. Toxicology and Applied Pharmacology.
2001;
176
(3)
:
145-52
.
View Article Google Scholar -
Albukhari
A.A.,
Gashlan
H.M.,
El-Beshbishy
H.A.,
Nagy
A.A.,
Abdel-Naim
A.B.,
Caffeic acid phenethyl ester protects against tamoxifen-induced hepatotoxicity in rats. Food and Chemical Toxicology.
2009;
47
(7)
:
1689-95
.
View Article Google Scholar -
Tabassum
H.,
Rehman
H.,
Banerjee
B.D.,
Raisuddin
S.,
Parvez
S.,
Attenuation of tamoxifen-induced hepatotoxicity by taurine in mice. Clinica Chimica Acta.
2006;
370
(1-2)
:
129-36
.
View Article Google Scholar -
Gao
F.F.,
Lv
J.W.,
Wang
Y.,
Fan
R.,
Li
Q.,
Zhang
Z.,
Tamoxifen induces hepatotoxicity and changes to hepatocyte morphology at the early stage of endocrinotherapy in mice. Biomedical Reports.
2016;
4
(1)
:
102-6
.
View Article Google Scholar
Comments
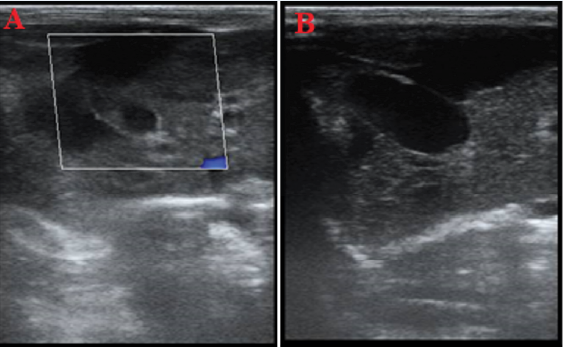
Article Details
Volume & Issue : Vol 10 No 12 (2023)
Page No.: 6075-6085
Published on: 2023-12-31
Citations
Copyrights & License

This work is licensed under a Creative Commons Attribution 4.0 International License.
Search Panel
Pubmed
Google Scholar
Pubmed
Google Scholar
Pubmed
Search for this article in:
Google Scholar
Researchgate
- HTML viewed - 1670 times
- PDF downloaded - 647 times
- XML downloaded - 46 times