Abstract
Introduction: Teucrium montanum has been traditionally used for immune system strengthening. However, T cell-related mechanisms of the Teucrium species remain poorly understood. In this study, we investigated the impact of T. montanum extracts and their fractions in stimulating proliferation and, more importantly, in differentiation into effectors and memory T cells.
Methods: The dried, aerial part of T. montanum was extracted in four different solvents: petroleum ether, dichloromethane, ethyl acetate, and methanol. A total of 67 fractions were subsequently collected from the dichloromethane extract in a stepwise gradient system of petroleum ether/acetone followed by methanol. An Alamar Blue assay and flow cytometry were employed to characterize the influence on T cell response by the plant extracts or fractions.
Results: The dichloromethane extract showed promotive effects on the proliferation and activation of total lymphocytes, and this was more prominent in CD8+ T cells. Among the 67 fractions from the dichloromethane extract, nine were found to demonstrate pro-proliferative activity. Further testing revealed that three methanolic or acetone subfractions out of the nine fractions were able to either promote effector/ effector memory differentiation of primed CD8+ T cells or skew the T cells into a central memory phenotype.
Conclusion: Our results suggest that T. montanum can drive proliferation of T cells and regulate the development of effector and memory populations, apparently corroborating the reported immunological benefits of T. montanum.
Introduction
Teucrium L., a large genus of perennial plants in the family Lamiaceae, is well known for its ability to prevent and cure a variety of illnesses. So far, more than 300 Teucrium species have been discovered globally. In particular, T. montanum L. has been used in traditional medicine since ancient times. Its numerous pharmacological activities are linked to a variety of purported beneficial effects regarding the inhibition of oxidative stress, proinflammatory response, allergenic reaction, microbial infection, insect infestation, and malignancy1, 2, 3, 4, 5, 6, 7. It has also been used to treat cutaneous and pulmonary tuberculosis8. For these reasons, it is considered to be a viable therapeutic option for various kinds of ailments and disorders, including cardiovascular disease, neurodegenerative disease, intestinal spasms, diarrhea, rheumatoid arthritis, inflammatory bowel disease, diabetes, and hyperlipidemia9, 10, 11. T. montanum acts as a healing herb and is capable of protecting from microbial infection, cirrhosis, and cancer, particularly in the respiratory and digestive tracts1, 12, 13, 14, 15. Notably, it has demonstrated strong antiproliferative activity, inducing early apoptosis of tumor cells as opposed to promoting apoptosis in the late stage as in the case of T. polium, for example2.
One of the most prominent effects of T. montanum is its ability to strengthen the immune system3, 16. T. montanum has commonly been used, often in the form of infusion, for its remarkable medicinal benefits17, 18. It is well known for being particularly rich in polyphenol antioxidants, which have a strong cytoprotective activity and have the capacity to raise immunity levels9, 19. Its methanolic extracts have been found to be capable of targeting tumors while preserving resting or activated immune cells in the peripheral blood, thereby strengthening the immune system’s response against cancer or reinforcing cancer immunotherapy20. Studies have also shown that T. montanum contains a significant amount of the essential minerals that are important for supporting and boosting immune function21. How immune cells are mechanistically involved in response to T. montanum stimulation remains unknown. Investigation of the immunological effects of other Teucrium species, such as T. polium and T. ramosissimum, has shown remarkable effects on lymphocytes or T cells with respect to their proliferative and activation capabilities22, 23, leading us to hypothesize that T. montanum may be able to stimulate the immune system during the priming phase of T cells. Based on the fact that the CD8+ subset represents the primary effector T cells participating in protective immunity, we chose to examine T. montanum extracts and chromatography fractions for their immunological activity in activation and differentiation of CD8+ T cells from naïve repertoire.
CD8+ T cells play a central role in cellular immunity, offering both primary and memory protection against infection and cancer24, 25, 26, 27. Antigenic stimulation through T cell receptors not only leads to proliferation and differentiation of naïve cells into effector cells, but also results in the formation of memory cells that retain the ability to mediate recall responses. Both effector and memory T cells can be generated simultaneously, while the memory population can emerge even before the response reaches its maximum, and can also be derived from a small fraction of early effector cells28. In order to combat microbial infection or cancer, which can be an overwhelming systemic, and often life-threatening, disease, the induction of memory T cells providing persistent immune surveillance is essential. Effector/effector memory T cells that recirculate between blood and nonlymphoid tissues are positioned to mobilize to sites of inflammation29, while central memory T cells (Tcm) primarily circulate and patrol the blood and lymph29; because of their potential to expand, differentiate, and self-renew, they are highly desirable for controlling systemic infection and cancer30, 31. In this study, lymphocytes from naïve mice were used to test various extracts and fractions obtained from T. montanum to assess their effects on cell proliferation and their potential to regulate T-cell differentiation.
Methods
General experimental procedures
Column chromatography was performed using a silica gel (200-300 mesh, Qingdao Marine Chemical Inc., China) and MCI gel HP-20 (75-150 μm, Mitsubishi Chemical Co., Ltd., Japan). Analytical high-performance liquid chromatography (HPLC) was performed on a Shimadzu LC-20AT instrument using a YMC C18 column (250 × 4.6 mm, 5 μm) with a flow rate of 1.0 mL/min, and with the gradient program of MeCN/H2O set to 10:90 (t = 0 min), 60:40 (t = 40 min), 60:40 (t = 45 min), 10:90 (t = 50 min), and 10:90 (t = 55 min). HPLC-grade acetonitrile, methanol, and other analytical grade reagents were purchased from Beijing Chemical Reagent Co., Ltd. (Beijing, China).
Plant Material
The plant Teucrium montanum L. was collected in Rtanj, Serbia (GPS: E 21.92658400, N 43.76136400), in August 2018. The plant material was identified by Prof. Z. Dajić Stevanović and taxonomically interpreted according to Euro+Med Plantbase and Flora of Serbia (Josifović 1970–1986) databases. An herbarium voucher (RS-030718-1) was deposited at the Department of Agricultural Botany in the Faculty of Agriculture, University of Belgrade.
Preparation of extracts and fractions
The aerial parts of T. montanum (10 kg) plants were air-dried, chopped, and extracted three times (2 h each) with 95% EtOH under reflux conditions. The EtOH extract was evaporated to almost dryness in vacuo, and the resulting residue (1040 g) was redissolved in MeOH, and mixed with Si gel (1500 g, 200–300 mesh). After removing MeOH, the dry mixture was extracted in a Soxhletanalytical-grade extractor (100 × 15 cm) successively with petroleum ether (PE, 8.0 L), dichloromethane (DM, 8.0 L), ethyl acetate (EA, 8.0 L), and methanol (MT, 8.0 L) under reflux conditions. The PE, DM, EA, and MT extracts were then evaporated to dryness under reduced pressure. The yields of the extracts were: PE, m = 187 g; DM, m = 90 g; EA, m = 105 g, and MT, m = 400 g. The DM portion (90 g) was subjected to Si gel column chromatography (80 × 8 cm, 200–300 mesh) and eluted with a petroleum ether/acetone (10:–-1:1, v/v) gradient system to yield thirteen fractions, IVA-A1~A8, IVA-B, IVA-C, IVA-D, IVA-E, and IVA-F, on the basis of TLC analysis. Fraction IVA-B (10 g), eluted with PE-acetone (8:1), was further fractionated by MCI gel HP-20 chromatography and eluted with a step gradient of MeOH-H2O (40:60, 60:40, 80:20, and 100:0 v/v) to give 10 subfractions, named IVA-B1~B10. Fraction IVA-C (9 g) was eluted with PE-acetone (6:1), fractionated by MCI gel HP-20 chromatography, and then eluted with a step gradient of MeOH-H2O (40:60, 60:40, 80:20, and 100:0 v/v) and acetone to yield 17 subfractions, named IVA-C1~C17. Similarly, fraction IVA-D (8 g) was eluted with PE-acetone (5:1), fractionated by MCI gel HP-20 chromatography, and eluted with a step gradient of MeOH-H2O (40:60, 60:40, 80:20, and 100:0 v/v) and acetone to yield 14 subfractions, named IVA-D1~D14. IVA-E (8.5 g) was eluted with PE-acetone (3:1), fractionated by MCI gel HP-20 chromatography, and eluted with a step gradient of MeOH-H2O (40:60, 60:40, 80:20, and 100:0 v/v) and acetone to yield 14 subfractions, named IVA-E1~E14. The last fraction, IVA-F (3.3 g), was eluted with PE-acetone (2:1), fractionated by MCI gel HP-20 chromatography, and eluted with a step gradient of MeOH-H2O (40:60, 80:20, and 100:0 v/v) to yield four subfractions, IVA-F1~F4 (Figure 1).
Cells and reagents
Primary splenocytes were isolated from 6–8-week-old C57BL/6 mice purchase from the Institute of Laboratory Animal Sciences (CAMS&PUMC, Beijing, China). Research was conducted in accordance with all institutional guidelines and ethics and approved by the Laboratories Institutional Animal Care and Use Committee of the Chinese Academy of Medical Sciences and Peking Union Medical College. All experimental protocols were reviewed and approved by the Institute of Materia Medica Animal Authorities (No. 00003375).
Alamar Blue (Cat. No. DAL1025) was purchased from Thermo (Waltham, USA) and antibodies for flow cytometry were purchased from BioLegend, eBioscience (San Diego, USA) or Signaling Technology (Danvers, USA) unless otherwise noted.
Alamar Blue assay
Splenocytes were re-suspended in RPMI medium (supplemented with 10% FBS, 1% penicillin and streptomycin, 10 mM HEPES, 1 mM glutamine, 1 mM sodium pyruvate and 55 μM β-mercaptoethanol) to prepare single cell suspensions and plated in 96-well U-button plates in concentrations of 2×105 cells/well under standard conditions (5% CO2, 95% humidity, 37 ℃). At 41 h post-stimulation, the cells were incubated with 5% Alamar Blue (v/v)32 for 7 h prior to measuring the fluorescence intensities at 566 nm excitation and 586 nm emission using an Enspire Microplate Reader (Perkin Elmer).
In vitro activation of lymphocytes
Splenocytes were incubated with ACK lysis buffer for 5 min to remove erythrocytes. Cells cultured in RPMI-1640, supplemented with 10% fetal bovine serum, penicillin (100 U/mL), streptomycin (100 mg/mL), and IL-2 (100 U/mL) (Peprotech) at 37 ℃ in 5% CO2, were stimulated with anti-CD3 (clone 145-2C11, 5 μg/ml, BioLegend) and anti-CD28 (clone 37.51, 2.5 μg/ml, BioLegend) mAbs for three days. Plant crude extracts or fractions were added to the culture at the beginning of experiments.
In vitro proliferation assay
Splenocytes were labeled with CFSE (10 μM, Sigma, USA) at 37 °C for 5 min. After removing excess CFSE, cells were cultured in a 96-well, round-bottom plate with 2×105 cells in complete RPMI 1640 medium supplemented with HEPES (10 mM), sodium pyruvate (1 mM), and 2-mercaptoethanol (55 μM) for 48 h before flow cytometry analysis.
Flow cytometry
Fluorescent dye-labeled antibodies against cell-surface markers CD8 (53-6.7), CD44 (IM7), CD62L (MEL-14), and CD69 (H1.2F3) were used for flow cytometry analysis. Prior to all flow cytometry staining, FcγIII/II receptors were blocked by incubating cells with anti-CD16/32 (2.4G2). All samples were acquired on a FACSVerse cytometer (Becton Dickson, USA) and data analysis was performed using FlowJo (TreeStar, USA).
Statistics
Statistical analyses and a Pearson’s correlation test were performed using GraphPad Prism 8 software. Comparisons among means of more than two groups were conducted with through one-way ANOVA with Tukey’s post-hoc test. P values less than 0.05 were considered statistically significant.
Results
Preparation of the four extracts from T. montanum and fractionation of the IVA-DM extract
Since T. montanum preparations usually consist of several dozen compounds, with few forming a sufficient majority to simply exert their effects singularly6, we tested different T. montanum extracts and fractions for their immunological effects on lymphocytes. As shown in the designed flowchart for the extraction and fractionation of T. montanum (Figure 1), four extracts of petroleum ether (IVA-PE), dichloromethane (IVA-DM), ethyl acetate (IVA-EA), and methanol (IVA-MT) from the aerial part of T. montanum were prepared (Figure 1A). Then, the lipid-soluble extract of IVA-DM was separated by silica gel column chromatography (Figure 1B) and fractionated by MCI gel HP-20 column chromatography to produce 67 fractions from all six crude fractions, IVA-A, IVA-B, IVA-C, IVA-D, IVA-E, and IVA-F (Figure 1C-G). The HPLC analyses of the bioactive extract IVA-DM and its chromatography fractions are shown in Figs. S1-S10.
T. montanum dichloromethane extract shows pro-proliferative activity
To determine the immunoactivity of T. montanum, we used Alamar Blue to assess the growth of lymphocytes upon stimulation with the various pre-fractionated extracts of the plant. Increased lymphocyte numbers were observed after 48 h of stimulation with IVA-DM (Figure 1). The CFSE-based proliferation assay confirmed that cells proliferated in response to IVA-DM, but not to any other extracts, such as IVA-PE, IVA-EA, or IVA-MT. These results suggest the presence of pro-proliferative ingredients in the T. montanum extracts.
We then screened all 67 fractions generated from chromatographically fractionated IVA-DM for pro-proliferative activities using the Alamar Blue assay and identified nine fractions with dose-dependent cell growth-promoting activity, as indicated in Figure 3. These included fractions separated into petroleum ether/acetone at ratios of 8:1 (IVA-B8, -B9, and -B10), 6:1 (IVA-C13, -C14, -C15, and -C16), 5:1 (IVA-D13), and 3:1 (IVA-E13), respectively. None of the other fractions, such as the IVA-A, IVA-D, and IVA-F groups, were found to have the same effect. It is possible that several IVA-DM fractions interacted together and each contributed to part of the overall pro-proliferative activity for T. montanum.
T. montanum extract IVA-DM activates naïve CD8+ T cells
CD8+ T cells play a central role in host immune response. Here, we evaluated how this type of cell responded to IVA-DM. CFSE-based analysis revealed that, compared to the CD8− T cell population, CD8+ T cells proliferated selectively in response to IVA-DM, while other extracts, such as IVA-EA, showed no potential to stimulate cell proliferation (Figure 4A). The data indicate that the T. montanum extract IVA-DM effectively stimulated CD8+ T cells to proliferate. We then assessed whether IVA-DM is capable of activating CD8+ T cells by measuring the expression of CD69, an early activation marker on the surface of T cells. Flow cytometric analysis showed a significant dose-dependent increase in CD69 expression and in the percentage of CD69+ cells in the CD8+ population 48 h after IVA-DM stimulation, whereas there was no CD69 upregulation by IVA-EA (Figure 4B). As CD44 is a cell surface adhesion molecule that plays an important role in T-cell activation, we then examined whether IVA-DM could stimulate CD44 expression. Figure 4C demonstrated that the percentage of cells expressing CD44 markedly increased in CD8+ T cells following IVA-DM treatment, unlike IVA-EA, reaffirming the activity of T. montanum IVA-DM on T-cell activation.
Some IVA-DM fractions enable a greater proportion of effector/effector memory differentiation of primed CD8+ T cells
We next explored whether the nine IVA-DM immunoreactive fractions could regulate the expression of CD44 and CD62L during the engagement of T-cell receptors. Among the nine fractions with proliferation-promoting activity, three of the 100% methanol-yielded fractions, IVA-B8, -B9, and -D13, and the only acetone fraction, IVA-C16, were able to upregulate CD44 expression dramatically (Figure 5A). Of the remaining five fractions collected from 100% methanol, IVA-B10 and -E13 failed to upregulate CD44 (Figure 5 A), while -C13, -C14 and -C15, which appeared to stimulate the highest cell proliferation, did not produce enough cells for analysis, presumably because of an inhibitory effect on cells activated by T-cell receptor engagement. Furthermore, we found that IVA-D13 induced a higher expression of CD62L, whereas the rest of the IVA-DM fractions did not demonstrate such effects (Figure 5 B).
CD44 and CD62L, as regulators of cell adhesion and migration, are essential in recruiting and activating effector and memory T cells. This prompted us to explore the role of these fractions in the differentiation of effector/effector memory (Teff/Tem) and central memory (Tcm) CD8+ T cells defined by CD44+CD62L− and CD44+CD62L+, respectively. At 10 μg/ml, IVA-B8, -B9, and -C16 led to a significantly increased percentage of Teff/Tem, while IVA-B10 and -E13 demonstrated no significant changes to the Teff/Tem population (Figure 5 C).
It has been demonstrated that there is an intermediate-stage cell type, referred to as CD44⁻CD62L⁻ pre-effector T cells, that are involved in differentiation from naïve to Teff/Tem33. It seems very likely that the reduction of pre-effector T cells (Tpe) could be a consequence of enhanced Teff/Tem differentiation. We thus sought to investigate the changes of Tpe following stimulation. Compared to α3/28 only, all Teff/Tem-promoting fractions (IVA-B8, -B9, and -C16 at 10 μg/ml) were able to drastically lower the Tpe subset. Furthermore, IVA-B10 and -E13 also inhibited Tpe, but not as much as the above four fractions. Thus, it is possible that the Tpe population reduced by IVA-B8, -B9, and -C16 could be related to increased Teff/Tem differentiation (Figure 5 C).
Certain IVA-DM fractions facilitate Tcm formation
In contrast to IVA-B8, -B9, and -C16, 10 μg/ml of IVA-D13 induced formation of more Tcm and a resulted in a substantially decreased proportion of Tpe (Figure 5 C). Thus, we reasoned that IVA-B10 and -E13 were unable to induce CD8+ T-cell differentiation at a lower dose, and therefore, that stimulation with a higher dose may be warranted. However, when the dose was increased to 30 μg/ml, obvious cytotoxicity was produced by IVA-B10 but not IVA-E13, which was then able to elevate the expression of both CD44 and CD62L (Figure 6 A). At this higher dose, IVA-E13 induced a significantly greater proportion of Tcm with a greatly reduced percentage of Tpe. Additionally, Tem was also substantially decreased (Figure 6 B). In sum, our findings demonstrate that the dichloromethane fractions IVA-B8, -B9, and -C16 were able to stimulate Tem differentiation, in contrast to IVA-D13 and -E13, which were capable of inducing Tcm formation.
Discussion
We demonstrate in the present study that the dichloromethane extract IVA-DM of T. montanum obtained from the Balkans was able to stimulate lymphocyte proliferation. The pro-proliferative effect could largely be attributed to a number of methanolic or acetone fractions of the dichloromethane extract that stimulate lymphoproliferation more effectively. More importantly, our study reveals previously unidentified immunological roles of T. montanum in T cell immunity, that is, the ability to modulate the effector and memory differentiation of T cells. A lower dose of fractions IVA-B8, -B9, -C16, (three out of the nine fractions with proliferation-promoting activity) enhanced CD8+ Teff/Tem differentiation. Fraction IVA-D13 at a lower dose and fraction IVA-E13 at a higher dose both promoted the development of Tcm. However, IVA-E13 also suppressed the formation of Teff/Tem when the dose increased, while IVA-D13 did not, which may suggest that IVA-E13 is capable of creating a state of balance between the two T cell subsets. Notably, T. montanum-derived essential oil has been shown to be inhibitory to proinflammatory T cells1, 34.
Indeed, CD8+ T cells become committed to proliferation and differentiation as an essential feature of the adaptive immunity, through which multiple distinct subsets are created to meet the requirements for the full execution of various specialized functions35. Increased effector memory differentiation could be advantageous, as recirculation of Teff/Tem cells through nonlymphoid tissues is essential for an immediate response because it leads to long-lived tissue memory T cells. Effector differentiation results in T cell acquisition of cytolytic activity; however, effector T cells also exert a profound pro-inflammatory influence on the host immune response. Although inflammation is necessarily essential for pathogen clearance, it can also cause tissue damage when present in excess36. Increased Tcm differentiation could thus be beneficial, as it is less cytotoxic and has higher proliferative and differentiation abilities than effectors in recall response37. Thus, it is feasible that elevated expression of CD62L promoted by IVA-E13 could enhance the quality of Tcm, leading to increased repopulating ability for the control of disease both systemically and locally in the tissue.
Some immunoactive substances have been implicated in many Teucrium species studied38, 39. Methanolic, aqueous, and total flavonoid extracts of T. ramosissimum have been found to induce splenocyte proliferation and enhance LPS-induced proliferative response22. Phenolic acids and flavonoids contained in T. polium and T. scordium methanolic extracts may have antimutagenic and proapoptotic effects, protecting lymphocytes from mitomycin C-induced genotoxicity40.
In general, both phenolic acids and flavonoids can be extracted efficiently with dichloromethane. Additionally, terpenoids are also found in dichloromethane extracts41, 42, and methanol/dichloromethane extraction of significant amounts of phenolic and terpene compounds from T. chamaedrys has been reported43. T. montanum essential oil, in particular, is known to be rich in terpenoids7, 44. Therefore, further investigation will be required to focus on identifying active compounds from the four fractions of the dichloromethane extract of T. montanum in order to facilitate development of a novel class of herbal medicines for T-cell based vaccine or immunotherapy.
Conclusions
We demonstrated in vitro lymphocyte proliferation-promoting activity of the dichloromethane fractions from T. montanum, and finding a number of its fractions to have considerable effector- and memory-differentiating effects on CD8+ T cells. Immunologically active compounds will need to be identified in the fractions that appear to skew T cells toward more Tcm than Tem.
Abbreviations
DM: dichloromethane, EA: ethyl acetate, MFI: mean fluorescence intensity, MT: methanol, PE: petroleum ether, RFU: relative fluorescence unit, Tcm: central memory T cell, Teff: effector T cell, Tem: effector memory T cell, Tpe: pre-effector T cell
Acknowledgments
None.
Author’s contributions
Conceptualization, S.Y, S.M., Z.D.S., and Q.Z.; methodology, S.M., J.L, Z.D.S., and Q.Z.; validation, Z.D.S, S.M., J.L., and Q.Z.; formal analysis, Z.D.S, S.M., J.L., and Q.Z.; investigation, S.M., J.L., Y.W., M.W., M.L., C.G., L.Z., Y.L., Y.L., and Z.D.S.; data curation, Z.D.S., S.M., and J.L.; writing—original draft preparation, J.L. and S.M.; writing—review and editing, Q.Z. and S.Y.; supervision, Q.Z. and S.Y.; funding acquisition, S.Y., N.A., N.T., Z.D.S, and Q.Z. All authors have read and agreed to the published version of the manuscript.
Funding
This work was supported by the National Natural Science Foundation of China (81871784, 82171822), The National High Technology Research and Development Program of China (2017YFE0112900), Beijing Key Laboratory of New Drug Mechanisms and Pharmacological Evaluation Study Project (BZ0150), and Yunnan Science and Technology Talent and Platform Program (202105AG070012).
Availability of data and materials
Data and materials used and/or analyzed during the current study are available from the corresponding author on reasonable request.
Ethics approval and consent to participate
Not applicable.
Consent for publication
Not applicable.
Competing interests
The authors declare that they have no competing interests.
References
-
Vukovic
N.,
Milosevic
T.,
Sukdolak
S.,
Solujic
S.,
Antimicrobial Activities of Essential Oil and Methanol Extract of Teucrium montanum. Evidence-Based Complementary and Alternative Medicine.
2007;
4
(s1)
:
17-20
.
View Article PubMed Google Scholar -
Stankovic
M.S.,
Curcic
M.G.,
Zizic
J.B.,
Topuzovic
M.D.,
Solujic
S.R.,
Markovic
S.D.,
Teucrium plant species as natural sources of novel anticancer compounds: antiproliferative, proapoptotic and antioxidant properties. International Journal of Molecular Sciences.
2011;
12
(7)
:
4190-205
.
View Article PubMed Google Scholar -
Stanković
M.S.,
Zlatić
N.M.,
Ethnobotany of Teucrium Species. Ethnobotany: CRC Press; 2019.CRC Press 2019.
Google Scholar -
Stankovic
M.S.,
Niciforovic
N.,
Topuzovic
M.,
Solujic
S.,
Total Phenolic Content, Flavonoid Concentrations and Antioxidant Activity, of The Whole Plant and Plant Parts Extracts fromTeucrium MontanumL. Var.Montanum, F.Supinum(L.) Reichenb. Biotechnology, Biotechnological Equipment.
2014;
25
(1)
:
2222-7
.
View Article Google Scholar -
Arcus
M.,
Schröder
V.,
Phytochemical resources on Thymus stojanovii degen. Species (labiatae). Annals of the Romanian Society for Cell Biology.
2017;
22
(1)
:
1-9
.
-
Pavela
R.,
Benelli
G.,
Canale
A.,
Maggi
F.,
Mártonfi
P.,
Exploring essential oils of Slovak medicinal plants for insecticidal activity: the case of Thymus alternans and Teucrium montanum subsp. jailae. Food and Chemical Toxicology.
2020;
138
:
111203
.
View Article PubMed Google Scholar -
Catinella
G.,
Badalamenti
N.,
Ilardi
V.,
Rosselli
S.,
De Martino
L.,
Bruno
M.,
The Essential Oil Compositions of Three Teucrium Taxa Growing Wild in Sicily: HCA and PCA Analyses. Molecules (Basel, Switzerland).
2021;
26
(3)
:
643
.
View Article PubMed Google Scholar -
Jarić
S.,
Ma\vcukanović-Jocić
M.,
Djurdjević
L.,
Mitrović
M.,
Kostić
O.,
Karad\vzić
B.,
An ethnobotanical survey of traditionally used plants on Suva planina mountain (south-eastern Serbia). Journal of Ethnopharmacology.
2015;
175
:
93-108
.
View Article PubMed Google Scholar -
\vCanadanović-Brunet
J.,
Djilas
S.M.,
Ćetković
G.,
Tumbas
V.,
Mandic
A.I.,
\vCanadanović
V.,
Antioxidant activities of different Teucrium montanum L. Extracts. International Journal of Food Science {&}amp; Technology.
2006;
41
(6)
:
667-73
.
View Article Google Scholar -
Djilas
S.M.,
Markov
S.L.,
Cvetković
D.D.,
Canadanović-Brunet
J.M.,
Cetković
G.S.,
Tumbas
V.T.,
Antimicrobial and free radical scavenging activities of Teucrium montanum. Fitoterapia.
2006;
77
(5)
:
401-3
.
View Article PubMed Google Scholar -
Vlase
L.,
Benedec
D.,
Hanganu
D.,
Damian
G.,
Csillag
I.,
Sevastre
B.,
Evaluation of antioxidant and antimicrobial activities and phenolic profile for Hyssopus officinalis, Ocimum basilicum and Teucrium chamaedrys. Molecules (Basel, Switzerland).
2014;
19
(5)
:
5490-507
.
View Article PubMed Google Scholar -
Redzic
S.,
Wild medicinal plants and their usage in traditional human therapy (Southern Bosnia and Herzegovina, W. Balkan). Journal of Medicinal Plants Research.
2010;
4
:
1003-27
.
-
Dinić
J.,
Novaković
M.,
Pešić
M.,
Potential for cancer treatment: natural products from the Balkans. 2020;
:
137-59
.
View Article Google Scholar -
Nikodijević
D.,
Milutinović
M.,
Cvetković
D.,
Stanković
M.,
\vZivanović
M.N.,
Marković
S.,
Effects of Teucrium Polium L. and Teucrium Montanum L. extracts on mechanisms of apoptosis in breast and colon cancer cells. Kragujevac. Journal of Science.
2016;
38
(38)
:
147-59
.
View Article Google Scholar -
Zivanovic
N.M.,
Stojanovic
Z.A.,
Cvetkovic
M.D.,
Milutinović
G.M.,
Stanković
S.M.,
Marković
D.S.,
Effects of Teucrium spp.: extracts on migratory potential and redox status of human colon SW-480 and breast MDA-MB-231 cancer cells. Kragujevac. Journal of Science.
2016;
(38)
:
161-72
.
View Article Google Scholar -
Jarić
S.,
Mitrović
M.,
Pavlović
P.,
Ethnobotanical Features of Teucrium Species. In: Stanković M, ed. Teucrium Species: Biology and Applications. Cham: Springer International Publishing; 2020:111-142.
.
View Article Google Scholar -
Zlatković
B.K.,
Bogosavljević
S.S.,
Radivojević
A.R.,
Pavlović
M.A.,
Traditional use of the native medicinal plant resource of Mt. Rtanj (Eastern Serbia): ethnobotanical evaluation and comparison. Journal of Ethnopharmacology.
2014;
151
(1)
:
704-13
.
View Article PubMed Google Scholar -
Janaćković
P.,
Gavrilović
M.,
Miletić
M.,
Radulović
M.,
Kolašinac
S.,
Stevanović
Z.D.,
Small regions as key sources of traditional knowledge: a quantitative ethnobotanical survey in the central Balkans. Journal of Ethnobiology and Ethnomedicine.
2022;
18
(1)
:
70
.
View Article PubMed Google Scholar -
Di Giacomo
S.,
Percaccio
E.,
Gull\`\i
M.,
Romano
A.,
Vitalone
A.,
Mazzanti
G.,
Recent Advances in the Neuroprotective Properties of Ferulic Acid in Alzheimer's Disease: A Narrative Review. Nutrients.
2022;
14
(18)
:
3709
.
View Article PubMed Google Scholar -
Stanković
M.S.,
Mitrović
T.L.,
Matić
I.Z.,
Topuzović
M.D.,
Stamenković
S.M.,
New Values of Teucrium species: in Vitro Study of Cytotoxic Activities of Secondary Metabolites. Notulae Botanicae Horti Agrobotanici Cluj-Napoca.
2015;
43
(1)
:
41-6
.
View Article Google Scholar -
Cindrić
I. Juranović,
Zeiner
M.,
Glamuzina
E.,
Stingeder
G.,
Elemental characterisation of the medical herbs Salvia officinalis L. and Teucrium montanum L. grown in Croatia. Microchemical Journal.
2013;
107
:
185-9
.
View Article Google Scholar -
Ben Sghaier
M.,
Krifa
M.,
Mensi
R.,
Bhouri
W.,
Ghedira
K.,
Chekir-Ghedira
L.,
In vitro and in vivo immunomodulatory and anti-ulcerogenic activities of Teucrium ramosissimum extracts. Journal of Immunotoxicology.
2011;
8
(4)
:
288-97
.
View Article PubMed Google Scholar -
Matic
S.,
Popovic
S.,
Baskic
D.,
Todorovic
D.,
Vukovic
N.,
Stankovic
M.,
Methanolic Extract of Teucrium Polium Exerts Immunomodulatory Properties in Human Peripheral Blood Mononuclear Cells. Serbian Journal of Experimental and Clinical Research.
2020;
23
(4)
:
345-51
.
View Article Google Scholar -
Hansen
S.G.,
Ford
J.C.,
Lewis
M.S.,
Ventura
A.B.,
Hughes
C.M.,
Coyne-Johnson
L.,
Profound early control of highly pathogenic SIV by an effector memory T-cell vaccine. Nature.
2011;
473
(7348)
:
523-7
.
View Article PubMed Google Scholar -
Masopust
D.,
Ha
S.J.,
Vezys
V.,
Ahmed
R.,
Stimulation history dictates memory CD8 T cell phenotype: implications for prime-boost vaccination. Journal of Immunology (Baltimore, Md.: 1950).
2006;
177
(2)
:
831-9
.
View Article PubMed Google Scholar -
Arunachalam
P.S.,
Charles
T.P.,
Joag
V.,
Bollimpelli
V.S.,
Scott
M.K.,
Wimmers
F.,
T cell-inducing vaccine durably prevents mucosal SHIV infection even with lower neutralizing antibody titers. Nature Medicine.
2020;
26
(6)
:
932-40
.
View Article PubMed Google Scholar -
Phan-Lai
V.,
Adoptive immunotherapy via CD4+ versus CD8+ T cells. Biomedical Research and Therapy.
2016;
3
(4)
:
16
.
View Article Google Scholar -
Obar
J.J.,
Lefrançois
L.,
Early signals during CD8 T cell priming regulate the generation of central memory cells. Journal of Immunology (Baltimore, Md.: 1950).
2010;
185
(1)
:
263-72
.
View Article PubMed Google Scholar -
Schenkel
J.M.,
Masopust
D.,
Tissue-resident memory T cells. Immunity.
2014;
41
(6)
:
886-97
.
View Article PubMed Google Scholar -
Berger
C.,
Jensen
M.C.,
Lansdorp
P.M.,
Gough
M.,
Elliott
C.,
Riddell
S.R.,
Adoptive transfer of effector CD8+ T cells derived from central memory cells establishes persistent T cell memory in primates. The Journal of Clinical Investigation.
2008;
118
(1)
:
294-305
.
View Article PubMed Google Scholar -
Cai
J.,
Zhao
J.,
Liu
D.,
Xie
H.,
Qi
H.,
Ma
J.,
Efficacy and Safety of Central Memory T Cells Combined With Adjuvant Therapy to Prevent Recurrence of Hepatocellular Carcinoma With Microvascular Invasion: A Pilot Study. Frontiers in Oncology.
2021;
11
:
781029
.
View Article PubMed Google Scholar -
Pham
P.V.,
Truong
K.D.,
Do
N.M.,
Nguyen
S.T.,
Vo
P.H.,
Sodium citrate inhibits proliferation and induces apoptosis of hepatocellular carcinoma cells. Biomedical Research and Therapy.
2020;
7
(3)
:
3659-66
.
View Article Google Scholar -
Nakajima
Y.,
Chamoto
K.,
Oura
T.,
Honjo
T.,
Critical role of the CD44lowCD62Llow CD8+ T cell subset in restoring antitumor immunity in aged mice. Proceedings of the National Academy of Sciences of the United States of America.
2021;
118
(23)
:
e2103730118
.
View Article PubMed Google Scholar -
Stankovic
M.S.,
Niciforovic
N.,
Topuzovic
M.,
Solujic
S.,
Total Phenolic Content, Flavonoid Concentrations and Antioxidant Activity, of The Whole Plant and Plant Parts Extracts from Teucrium Montanum L. Var. Montanum, F. Supinum (L.) Reichenb. Biotechnology & Biotechnological Equipment.
2011;
25
(1)
:
2222-7
.
View Article Google Scholar -
Masopust
D.,
Schenkel
J.M.,
The integration of T cell migration, differentiation and function. Nature Reviews. Immunology.
2013;
13
(5)
:
309-20
.
View Article PubMed Google Scholar -
Hufford
M.M.,
Kim
T.S.,
Sun
J.,
Braciale
T.J.,
Antiviral CD8+ T cell effector activities in situ are regulated by target cell type. The Journal of Experimental Medicine.
2011;
208
(1)
:
167-80
.
View Article PubMed Google Scholar -
Geginat
J.,
Lanzavecchia
A.,
Sallusto
F.,
Proliferation and differentiation potential of human CD8+ memory T-cell subsets in response to antigen or homeostatic cytokines. Blood.
2003;
101
(11)
:
4260-6
.
View Article PubMed Google Scholar -
Sargin
S.A.,
Büyükcengiz
M.,
Plants used in ethnomedicinal practices in Gulnar district of Mersin, Turkey. Journal of Herbal Medicine.
2019;
15
:
100224
.
View Article Google Scholar -
Martino
L. De,
Coppola
R.,
Feo
V. De,
Caputo
L.,
Fratianni
F.,
Nazzaro
F.,
Essential Oils Diversity of Teucrium Species. In: Stanković M, ed. Teucrium Species: Biology and Applications. Cham: Springer International Publishing; 2020:179-210.
.
View Article Google Scholar -
Milošević-Djordjević
O.,
Radović Jakovljević
M.,
Marković
A.,
Stanković
M.,
Ćirić
A.,
Marinković
D.,
Polyphenolic contents of Teucrium polium L. and Teucrium scordium L. associated with their protective effects against MMC-induced chromosomal damage in cultured human peripheral blood lymphocytes. Turkish Journal of Biology.
2018;
42
(2)
:
152-62
.
PubMed Google Scholar -
Chizzola
R.,
Volatile compounds from some wild growing aromatic herbs of the Lamiaceae from southern France. Plant Biosystems.
2006;
140
(2)
:
206-10
.
View Article Google Scholar -
Panicker
N.G.,
Balhamar
S.O.,
Akhlaq
S.,
Qureshi
M.M.,
Rizvi
T.S.,
Al-Harrasi
A.,
Identification and Characterization of the Caspase-Mediated Apoptotic Activity of Teucrium mascatense and an Isolated Compound in Human Cancer Cells. Molecules (Basel, Switzerland).
2019;
24
(5)
:
977
.
View Article PubMed Google Scholar -
Elmastas
M.,
Erenler
R.,
Isnac
B.,
Aksit
H.,
Sen
O.,
Genc
N.,
Isolation and identification of a new neo-clerodane diterpenoid from Teucrium chamaedrys L. Natural Product Research.
2016;
30
(3)
:
299-304
.
View Article PubMed Google Scholar -
Bezić
N.,
Vuko
E.,
Dunkić
V.,
Rušscić
M.,
Blazević
I.,
Burcul
F.,
Antiphytoviral activity of sesquiterpene-rich essential oils from four croatian teucrium species. Molecules (Basel, Switzerland).
2011;
16
(9)
:
8119-29
.
View Article PubMed Google Scholar
Comments
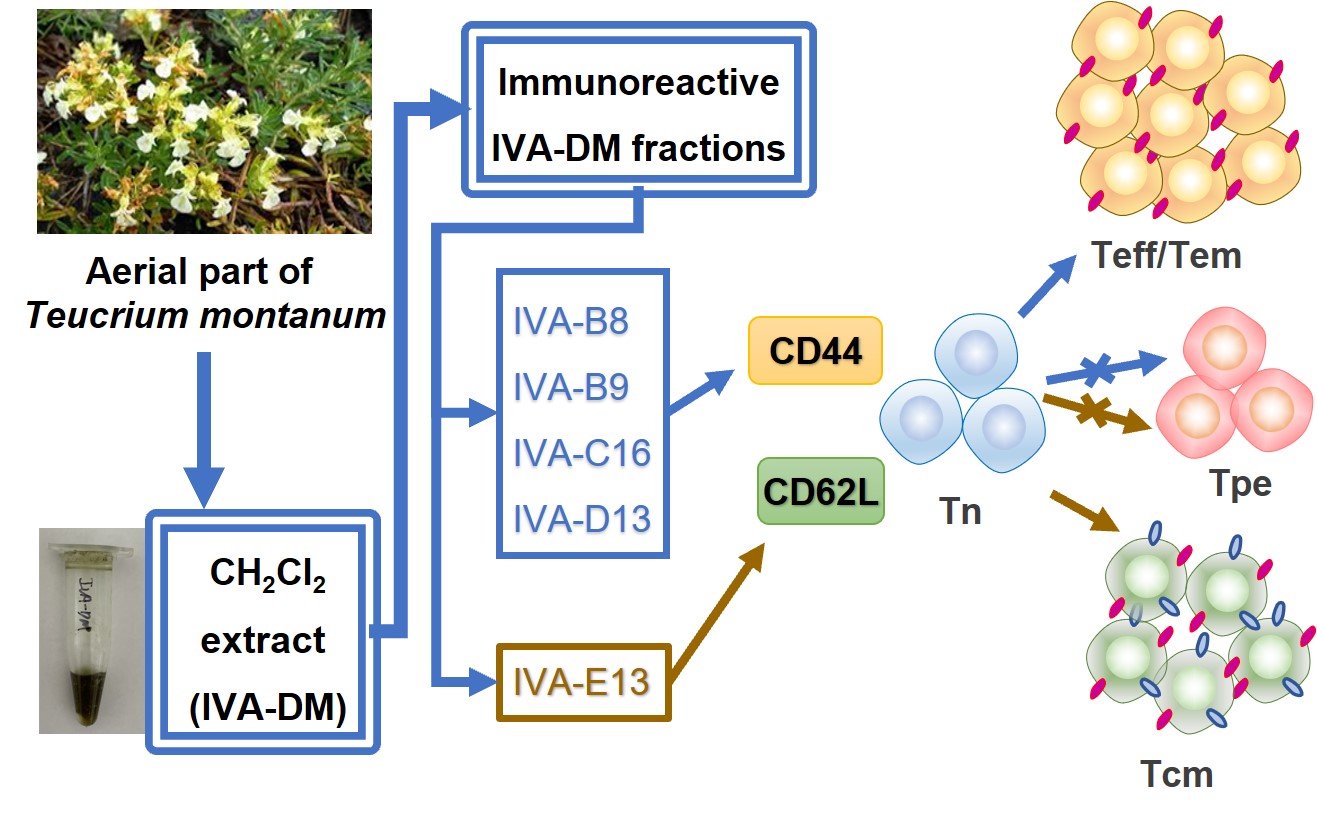
Article Details
Volume & Issue : Vol 10 No 11 (2023)
Page No.: 6023-6034
Published on: 2023-11-30
Citations
Copyrights & License

This work is licensed under a Creative Commons Attribution 4.0 International License.
Search Panel
Pubmed
Google Scholar
Pubmed
Google Scholar
Pubmed
Google Scholar
Pubmed
Google Scholar
Pubmed
Google Scholar
Pubmed
Google Scholar
Pubmed
Google Scholar
Pubmed
Google Scholar
Pubmed
Google Scholar
Pubmed
Google Scholar
Pubmed
Google Scholar
Pubmed
Google Scholar
Pubmed
Google Scholar
Pubmed
Search for this article in:
Google Scholar
Researchgate
- HTML viewed - 1205 times
- PDF downloaded - 405 times
- XML downloaded - 26 times