Abstract
Introduction: In regenerative medicine, human adipose tissue–derived mesenchymal stem cells (hadMSCs) have exhibited tremendous promise as an efficient approach to chronic cardiovascular diseases. Noble effects of mesenchymal stem cells (MSCs) have been acknowledged to contribute to not only cellular engraftment and response to the site of injury but also paracrine action via the release of extracellular vesicles, notably exosomes. This study aimed to investigate the effects of exosomes from hadMSCs (hadMSC-Exo) on the angiogenesis of endothelial cells.
Methods: Firstly, hadMSCs were characterized and cultured at a density of 500,000 cells in exosome-free medium for 48 hours. The conditioned medium was ultracentrifuged for exosome isolation. Obtained exosomes were characterized with the expression of CD9, CD63, and CD81 markers by flow cytometry and imaged with a TEM microscope. Exosomes were added to a culture medium of human umbilical vein endothelial cells (HUVECs) to understand the effects of exosomes on HUVECs. The expression of some angiogenic genes in HUVECs was identified by RT-qPCR.
Results: The concentration of total protein in isolated exosomes by Bradford assay was 0.51 ± 0.04 mg/mL. Exosomes were successfully isolated and characterized by their morphology and immunophenotype. The results showed that exosomes significantly increased the mRNA expression of TGF (11.56 ± 2.03, P < 0.05), Flk-1 (4.04 ± 0.01, P < 0.05), VE-cadherin (7.25 ± 2.30, P < 0.05), and CD31 (3.02 ± 1.13, P < 0.05).
Conclusion: This study suggested that exosomes promoted the angiogenesis of endothelial cells in vitro.
Introduction
According to the World Health Organization, cardiovascular diseases are currently the leading cause of death globally. Stem cell therapies are gradually becoming key players in regenerative medicine. In recent decades, MSCs have made their mark in regenerative medicine applications. MSCs were first identified and characterized over 30 years ago, with over 55,000 papers currently on the subject1. These subpopulations of adult somatic stem cells have a wide anatomical distribution in the stroma of many tissues, including the bone marrow, adipose tissue, umbilical cord, skin, lung, dental pulp, and synovial membrane, which could undergo different cell lineages, such as adipocytes, chondrocytes, myocytes, osteoblasts, and neurocytes2. Despite MSCs being relatively rare within these tissues, they are known for their capacity for in vitro amplification and self-renewal. Due to their low expression of antigen-presenting molecules, MSCs elicit low immunogenicity and profound immunomodulatory properties. Moreover, several pieces of evidence have demonstrated that MSCs play pivotal roles in prohibiting apoptosis, inducing angiogenesis and cellular proliferation, and hindering the onset and progression of inflammation during tissue regeneration3. In terms of cardiovascular diseases, MSCs substantially exert immunoregulatory abilities, antifibrotic effects, and neovascularization traits4.
Remarkably, several studies have demonstrated that MSCs might desire not to repair organ defects by differentiating into the aimed tissue site, but rather accelerate regulatory roles of key biologic processes via regulatory and trophic secreting profiles5. Over the past decade, the emphasis has shifted toward utilizing the MSCs’ ability to manufacture factors and cytokines that provoke innate tissue repair and modulate inflammation and immune responses1. The novel regulatory mechanism of MSCs is believed to be beneficial to the divergent secretome of growth factors, cytokine secretion, cell–cell interaction, miRNA, and non-coding RNA transmission that are compacted within secreted extracellular vesicles (EVs), notably exosomes6. Exosomes (approximately 50 – 150 nm) are the smallest among Evs, which are nonviable yet bioactive vesicles encased by a lipid bilayer. The surface exosome comprises an evolutionarily conserved set of proteins including annexins, which are vital for transport; tetraspanins (CD9, CD63, and CD81) for cell targeting; and heat-shock proteins (HSP60, HSP70, and HSP90), ALIX, and tumor susceptibility gene 101 (TSG101), which are engaged in exosomal biogenesis from endosomes. They also manifest unique tissue type–specific proteins that reflect their cellular sources that can be selectively devoured by adjacent or distant cells far from their release, reprogramming the recipient cells into their bioactive compounds3. The molecular constituent of exosomes is not an exact representation of the cell at a smaller scale. Exosomes are enriched with specific RNAs or proteins, whereas other molecules are absent, suggesting the existence of a specialized regimen directing the loading of molecules into exosomes7. Exosomes can transmit information to target cells by binding to the cell surface by ligand, endocytosis, and direct fusion of extracellular with plasma membranes. Several reports have shown that the MSC secretome can drive organ healing by evoking a shift from proinflammatory to anti-inflammatory cytokine manufacture at the site of injury8. Such prospects of these mediators encompass immunomodulation and anti-inflammatory activities involving a wide spectrum of medical treatments, including graft versus host diseases after allogeneic transplantation, inflammatory disorders (e.g., allergy, asthma), autoimmune diseases (e.g., type 1 diabetes, rheumatoid arthritis, systemic lupus), and chronic wounds (e.g., diabetic wounds, ischemic wounds, ulcers).
Exosomes derived from MSCs (MSC-Exo) have been shown to elicit positive effects on cancers by inhibiting cell proliferation and metastasis9. Furthermore, recent studies have demonstrated the role of MSC-Exo on viral infectious diseases by suppressing cytokine storms, such as in Covid-1910. In addition, exosomes have been exploited for targeted drug delivery and as gene carriers in regenerative medicine11.
These reports highlight the blooming of cell-free and secretome-based therapies and that their advantages overcome some intrinsic risks of stem-cell-based applications. Throughout the process of cell expansion, cells might undergo DNA damage or mutation, which might change the behavior of transplanted cells12. This may include transdifferentiation into unseemly cell types13 or tumorigenicity potential14. Other considerations associated with transplantation to the patient’s body are immune compatibility, the transmission of infections, and proarrhythmic side effects15, 16. Moreover, the delivery of MSCs into the engraftment site might cause microvasculature hindrance compared to exosome-based approaches. In contrast, the nano-sizes of exosomes permit efficient travel into bloodstreams and throughout capillaries to sites of injury. Mass manufacturing of exosomes could be performed under controlled laboratory circumstances using custom-made cell lines, which provide a convenient supply of bioactive components. Moreover, biological products obtained for therapeutic purposes might be altered to achieve desired cell-specific outcomes. In particular, off-the-shelf secretome therapies could be instantly accessible for the cure of acute conditions such as cerebral ischemia, myocardial infarction, or military trauma16. Regarding long-term preservation, exosomes do not require the use of potentially harmful cryopreservative chemicals with loss of product potency17.
Notably, hadMSCs are appearing as one of the major sources of MSCs, which are known for their abundance. They require minimally invasive surgical interference and yield a higher number of cells and higher cell viability, as well as holding the most significant secretory function compared to MSCs from the bone marrow and umbilical cord, the other two common sources18, 19. Hence, hadMSCs have been safe, with limited risks or adverse effects in autologous or autologous transplantation in numerous cell-based therapies1. HadMSCs have been provided as promising remedies for various chronic diseases such as neurodegenerative diseases20, allergies21, and autoimmune diseases22. Interestingly, hadMSCs have been demonstrated to exert the most significant secretory function, notably exosomes among other evaluated sources of MSCs including the bone marrow and umbilical cord that have gained the most attention23.
In cardiovascular diseases, hadMSCs have manifested potential therapeutic applications for ischemic tissue–related diseases via exosome secretion of a robust profile of angiogenic signaling factors. This cell-free medical approach is a paramount prospect in mediating intercellular communication via cell‐to‐cell transport of proteins and RNA among cells, including immune cells and endothelial cells. MSC-Exo can protect myocardial cells from apoptosis, inflammation, fibrosis, and enhanced angiogenesis, thereby lessening acute myocardial ischemic injury24. Remarkably, clinical experiments on hadMSCs have reported enhanced cardiac function, which may be mainly due to the paracrine effect of secreting cytokines instead of through cardiac regeneration or differentiation1, 25. In other words, exosomes can shield the ischemic myocardium from apoptosis26 and stimulate angiogenesis27. Zhang et al. elucidated the miRNA profiles of hadMSC-Exos and indicated that a total of 148 and 154 known miRNAs were identified, which function in repressing their target mRNAs in recipient cells in vitro28. Notably, miRs are claimed as key regulators of endothelial cell function and are especially important modulators of angiogenesis23. Furthermore, proteomic analysis of adMSC-Exos revealed 1,466 proteins that are involved in various cell functions, especially proangiogenic signaling–associated proteins7, 29. The most direct and efficient embodiment of physiological functions, exosomal proteins play an equally essential role in signal transduction and regulation. Notably, several putative paracrine effectors of angiogenesis include platelet-derived growth factor, epidermal growth factor, fibroblast growth factor, and most notably nuclear factor kappa B (NFκB)-signaling pathway proteins enriched in exosomes30, 31.
Concerning angiogenesis, the regulation of blood vessel formation is critical for several processes, notably wound healing, tissue repair, reproduction, and development1. Angiogenesis is a form of neovascularization that constructs a network of thin tubules enduring a series of morphogenetic events involving sprouting, splitting, and remodeling. However, angiogenesis is relatively rare in adults32. Angiogenesis is a hallmark of many ischemic diseases, such as coronary artery disease, stroke, and chronic wounds. Several studies have revealed the effect of MSC secretome on key steps in angiogenesis. Furthermore, rapid activation of angiogenesis might determine the successful survival of transplanted cells, which initiates the formation of new blood vessels from pre-existing ones, thereby providing an immediate vascular supply of newly formed tissues to deliver oxygen and nutrients, eliminate waste products, and prevent necrosis33. Hence, MSC-EV therapeutics based on exosome delivery seem to hold the greatest prospect in regenerative medicine, and angiogenesis is a major area for novel therapeutic approaches to diseases. During the process of angiogenesis, the stimulation of angiogenic factors to the receptor initiates many cellular signaling pathways and molecules regarding growth and stabilization factors.
Given these advantages and the promising potential of exosomes, we hypothesized that exosomes from hadMSCs might promote angiogenesis to treat ischemia.
Methods
Human mesenchymal stem cells derived from adipose tissue (hadMSCs) and human umbilical vein endothelial cells (HUVECs)
hadMSCs and HUVECs were provided by SCI Cellbank (Stem Cell Institute, University of Science, HCMC, VN).
hadMSC cell culture and characterization
Cell culture
Cells were seeded in 75-cm2 cell culture flasks at a density of 0.5 x 106 cells and cultured with MSCCult MV media (exosome-free medium for MSC expansion, Regenmedlab, VN). The cells were cultured in an incubator at 37 ºC with 5% CO2. Cells were maintained in passage 3 to passage 7 and passaged at a split ratio of 1:3 after reaching approximately 80–90% confluence. Fresh medium was changed every two days, whereas cultured medium (conditioned culture medium) was stored at 4 ºC for exosome isolation.
Immunophenotyping
hadMSCs were harvested and incubated with FIT-C-conjugated antibodies against CD14 (00007, Miltenyi Biotec, Germany), CD34 (00001, Miltenyi Biotec, Germany), HLA-DR (00007, Miltenyi Biotec, Germany), phycoerythrin (PE)-conjugated antibodies against CD73 (00002, Miltenyi Biotec, Germany), CD90 (00003, Miltenyi Biotec, Germany), PerCP-conjugated antibodies against CD105 (00003, Miltenyi Biotec, Germany), CD19 (347544, BD Biosciences, USA), and APC-conjugated antibodies against CD45 (00005, Miltenyi Biotec, Germany), and then the excess antibodies were washed off. At least 1 x 105 cells per sample were acquired and analyzed. For result analysis, a BD FACSCalibur flow cytometer (BD Biosciences, USA) was used to study the haMSCs. Cell size plots were analyzed using BD CellQuest Pro software (BD Biosciences, USA) using forward scatter (FSC, an indirect measurement of size) and side scatters (SSC, a measurement of granularity).
In vitro differentiation
hadMSCs were induced to in vitro differentiated adipocytes, chondrocytes, and osteoblasts. Cells were seeded at 5 x103 cells into each well of a 96-well dish (SPL Life Science, Korea). Cells were then allowed to grow into 70–80% confluence and cultured in adipogenesis differentiation media: StemPro® Adipogenesis Differentiation Kit (Thermo Fisher Scientific, USA), chondrogenesis differentiation media—StemPro® Chondrogenesis Differentiation Kit (Thermo Fisher Scientific, USA), and osteogenesis differentiation media—StemPro® Osteogenesis Differentiation Kit (Thermo Fisher Scientific, USA). The medium was changed every 5 – 7 days. To evaluate their differentiating ability into osteoblasts, chondrocytes, and adipocytes, cells were stained with Alizarin Red as an indicator of calcium ion accumulation on day 21, with Alcian Blue as an indicator of extracellular proteoglycan matrix on day 14, and with Oil Red as an indicator of intracellular lipid droplets on days 7 – 14, respectively.
Exosome isolation and characterization
Exosome isolation
The procedure of exosome isolation from conditioned culture medium followed a five-step process previously described34. Firstly, cells were removed by centrifuging at 300x g for 10 minutes, then the supernatant was centrifuged at 2,000x g for 10 minutes to eliminate dead cells, then at 10,000x g for 30 minutes to exclude any cell debris. Thereafter, the supernatant was ultracentrifuged at 100,000x g for 70 minutes. The crude exosome pellet was carefully obtained and subsequently washed in PBS- to expel any contaminating proteins and centrifuged again at 100,000x g for 70 minutes to obtain the exosome pellet. Exosomes were resuspended in PBS-. The ultracentrifugation process was performed thoroughly at 4 ºC.
Bradford assay
The yield of exosomes was relatively measured by Bradford assay. Briefly, exosomes were stained with Coomassie Blue G-250 (Sigma, USA), and a standard BSA dilution curve (0, 0.25, 0.5, 0.75, 1, 1.5, 2 mg/ml) was constructed to calculate the protein quantity. The absorption result was read at 595 nm. The measurement was assessed by Beckman Coulter DTX 880 Multimode Detector (Beckman, Germany).
Identification of exosome immunotype by bead-assisted flow cytometry
The expression of exosome surface markers was conducted by flow cytometry following the standard instruction of Théry et al.34. Due to their nano size, exosomes were attached to a 3.9-µm Aldehyde/Sulfate Latex bead (A37304, ThermoFisher, USA) to adopt flow cytometry analysis. Briefly, approximately 10 µg of purified exosomes were incubated with 1 µL of latex beads for 15 minutes at room temperature (RT). PBS- was then added to obtain a 1-mL mixture, followed by incubation for 2 hours at RT. The mixture was added to 110 µL 1M Glycine (Sigma-Aldrich, USA) and mixed by gentle vortex, then rested for 30 minutes at RT. Thereafter, centrifugation was performed at 4,000 rpm for 3 minutes at RT. After discarding the supernatant, the bead pellet was suspended in 1 mL of staining buffer following centrifugation at 4,000 rpm for 3 minutes at RT. The pellet was then suspended in 200 µL of staining buffer and divided into four microcentrifuge tubes (SDL Life Science, Korea). Thereafter, exosomes were stained with FITC-conjugated antibodies against CD9 (AB_10667772, Thermo Fisher Scientific, USA), PE-conjugated antibodies against CD63 (AB_2572565, Thermo Fisher Scientific, USA), and APC-conjugated antibodies against CD81 (AB_11150235, Thermo Fisher Scientific, USA) for 30 minutes at 4 ºC, followed by washing twice with 200 µL of staining buffer. Exosomes were then resuspended in 200 µ PBS- into flow cytometry tubes (BD Biosciences, USA). Samples of unstained exosomes were used as a negative control. For result analysis, a BD FACSCalibur flow cytometer (BD Biosciences, USA) was used to study the exosomes. Exosome size plots were analyzed by BD CellQuest Pro software (BD Biosciences, USA) using FSC and SSC to analyze the separated beads and clumps of beads. Both types were gated by comparing FSC/SSC diagrams of beads of known size. Cell debris was excluded by gates in the right-angle scatter versus FSC diagrams and by PI fluorescence, which was read using a 610/20-nm band-pass filter.
Cell culture of HUVECs
Cells were seeded in a 6-well dish (SPL Life Science, Korea) with around 100,000 cells in each well and cultured with EGM media (Cell Applications, Inc, CA, USA) in an incubator at 37 ºC with 5% CO2. After reaching approximately 80 — 90% confluence, cells were further used as an experimental model of angiogenesis. Cells were observed under an inverted microscope (Carl Zeiss, Germany), and images were acquired using AxioVision Release 4.8.0 software (Carl Zeiss, Germany).
Evaluation of effects of exosomes on angiogenic gene expression in HUVECs
The HUVECs were seeded at a density of 1 × 104 cells/well in an endothelial cell growth medium (EGM medium) with 20 μg/mL of exosomes for 24hrs. Cells were then collected to isolate total RNA for gene expression evaluation. The HUVECs cultured in EGM medium without exosomes were used as control.
The expression of angiogenic genes included vascular endothelial cadherin (VE-cadherin), platelet endothelial cell adhesion molecule-1 (PECAM-1/CD31), Von Willebrand factor (Vwf), Fms-like tyrosine kinase 1 (Flt-1), vascular endothelial growth factor receptor 2 (VEGFR-2) or fetal liver kinase 1 (Flk-1), transforming growth factor (TGF-β), hepatocyte growth factor (HGF), angioietin-1 (ANG1), and angiopoietin-2 (ANG2) (Table 1). These angiogenesis-related mRNAs were identified by SYBR Green dye-based RT-qPCR to evaluate the exosome effect on angiogenesis in the HUVEC model.
HUVECs from the two experimental groups were lysed with 250 µL of EasyBlue Total RNA Extraction Kit (Intron, Korea), and cell lysate was then mixed with 50 µL of Chloroform (Sigma-Aldrich, USA), followed by centrifugation at 13,000 rpm for 5 minutes at 4 ºC. After around 150 µL of supernatant was carefully removed into a new tube, 150 µL of isopropanol (Sigma, MO) was added into the tube and incubated for 30 minutes at 4 ºC following centrifugation at 13,000 rpm for 5 minutes at 4 ºC. The supernatant was aspirated to collect the RNA, which was washed twice with ice-cold Ethanol 75º (Sigma-Aldrich, MO, USA). The RNA sample was collected and stored in DEPC-treated water at -20 ºC for a week. The RNA concentration and purification were determined photometrically.
Isolated RNA was kept on ice before RT-qPCR with specific primers using Luna Universal One-Step Reaction Mix (E3005, NEB BioLabs, England). Approximately 100 ng of total RNA was added into the assay mix per reaction. The mRNA quantitation was normalized to an endogenous control mRNA. The following primers were designed for the amplification of target mRNAs. The thermocycling conditions used were as follows: 55 ºC for 10 minutes, 95 ºC for 1 minute; followed by 40 cycles of 95 ºC for 10 seconds, followed by a final extension of 60 ºC for 30 seconds. Peaks of the melting curve were subsequently recorded: 95 ºC for 15 seconds, 60 ºC for 15 seconds, 60 – 95 ºC for 20 minutes, and 95 ºC for 15 seconds.
Isolated RNA underwent real-time SYBR Green qRT-PCR amplification. Levels of mRNA were determined using Universal One-Step Reaction Mix (E3005, NEB BioLabs, England) and measured by Mastercycler® ep realplex software (QIAGEN, USA). The cycle threshold (Ct) value, at which the fluorescence signals meet a threshold set within the logarithmic phase, was extracted, and 2-ΔΔCt was calculated to determine relative quantification. Relative mRNA levels of the genes were normalized to GAPDH.
No | Gene | Length (bp) | Primer sequences (5'-3') | NCBI |
---|---|---|---|---|
1 | GAPDH | 131 | F: GTCTCCTCTGACTTCAACAGCG | NM_001357943.2 |
R: ACCACCCTGTTGCTGTAGCCAA | ||||
2 | TGF-beta | 70 | F: CATGGAGCTGGTGAAACGGA | NM_011577.2 |
R: GGCGAGCCTTAGTTTGGACA | ||||
3 | Flk -1 | 132 | F: GGAACCTCACTATCCGCAGAGT | NM_002253.4 |
R: CCAAGTTCGTCTTTTCCTGGGC | ||||
4 | Flt-1 | 118 | F: CCTGCAAGATTCAGGCACCTATG | NM_001160030.2 |
R: GTTTCGCAGGAGGTATGGTGCT | ||||
5 | Vwf | 157 | F: CCTTGAATCCCAGTGACCCTGA | NM_000552.5 |
R: GGTTCCGAGATGTCCTCCACAT | ||||
6 | VE Cadherin | 112 | F: GAAGCCTCTGATTGGCACAGTG | NM_001795.5 |
R: TTTTGTGACTCGGAAGAACTGGC | ||||
7 | CD31 | 133 | F: AAGTGGAGTCCAGCCGCATATC | NM_000442.5 |
R: ATGGAGCAGGACAGGTTCAGTC | ||||
8 | ANG1 | 150 | F: CAACAGTGTCCTTCAGAAGCAGC | NM_001314051.2 |
R: CCAGCTTGATATACATCTGCACAG | ||||
9 | ANG 2 | 139 | F: ATTCAGCGACGTGAGGATGGCA | NM_001386337.1 |
R: GCACATAGCGTTGCTGATTAGTC | ||||
10 | HGF | 102 | F: GAGAGTTGGGTTCTTACTGCACG | NM_001010932.3 |
R: CTCATCTCCTCTTCCGTGGACA |
Statistical analysis
All data from in vitro experiments are expressed as the mean ± standard deviation (SD) and obtained from at least three independent experiments. Statistical analyses were performed using a two-tailed Student’s t-test (GraphPad Software, Sorrento Valley, CA, USA) to determine significant differences between groups. P < 0.05 was considered statistically significant.
Results
Characterization of hadMSCs
Firstly, the cultured population of hadMSCs showed typical morphology in that cells adhered to the surface of the plastic culture flask and manifested a spindle-shaped fibroblast-like morphology. Secondly, the cytochemical staining results revealed the differentiation potential of MSCs into osteoblasts, chondrocytes, and adipocytes (Figure 1). In the first 5 days, cells were enlarged in volume, with small spheres within them, indicating that cells in the progression of differentiation into adipocytes until day 14 were those stained with Oil Red dye to clearly determine the formation of lipid droplets. At the same time, staining cells with Alcian Blue was incorporated into substituents of the extracellular matrix such as proteoglycan. After 21 days of induction of osteogenesis differentiation, cells stained with Alizarin Red revealed the formation of calcium crystallization of mineralized nodules, which were shown clearly in a reddish color.
Thirdly, the flow cytometry analysis determined that the immunophenotype of MSCs expressed strongly positive for MSC-specific markers (>95%), including CD73 (99.36%), CD90 (99.46%), and CD105 (95.41%). On the other hand, CD14 (0.33%), CD19 (0.15%), CD34 (1.36%), CD45 (0.51%), and HLA-DR (0.39%) antigens were merely deficiently expressed (<2%; Figure 1). Hence, these results confirmed that the experimental hadMSCs had typical characteristics of MSCs.
Characterization and quantification of hadMSC-Exo
Under TEM, the hadMSC-Exo were spherical with a typical cup shape and bilayer membrane vesicles of different sizes (Figure 2). The diameter of exosomes was around 150 nm. The bead-assisted flow cytometry analysis confirmed the presentative markers of exosomes of CD9, CD63, and CD81. Based on the total amount of stained exosome beads, CD9+, CD63+, and CD81+ exosomes made up 56.5 ± 6.08%, 87.82 ± 2.29%, and 56.36 ± 16.7% of all exosomes identified, respectively (Figure 2). The co-expression of the three markers was shown at 26.37 ± 5.09%. Additionally, the Bradford assay showed that exosomes were isolated at the concentration of 0.51 ± 0.04 µg/mL (the amount of total protein in μg normalized to mL conditioned medium used for exosome isolation; n = 4). Hence, these results indicated that exosome isolation from hadMSCs was successfully performed.
HadMSC-Exo promoted angiogenesis of HUVECs in vitro
To elucidate the mechanism of the enhancing effect of hadMSC-Exo treatment on angiogenesis, RT-qPCR analysis suggested that compared with the control group, HUVECs under exosome treatment (20 µg/mL) for 24 hours were associated with a significantly greater chance of TGF (11.56 ± 2.03, P < 0.05), Flk-1 (4.04 ± 0.01, P < 0.05), VE-cadherin (7.25 ± 2.30, P < 0.05), and CD31 (3.02 ± 1.13, P < 0.05; Figure 3).
Discussion
In this study, we aimed to isolate and characterize hadMSC-Exo to initially investigate its proangiogenic effect. We focused on evaluating the expression of angiogenesis-related genes. The results revealed that compared with the untreated control group, the hadMSC-Exo-treated group significantly promoted the angiogenesis of HUVECs. The RT-qPCR data illustrated that hadMSC-Exo remarkably upregulated the mRNA expression of VEGFR-2, TGFβ, VE-cadherin, and CD31 in HUVECs (P < 0.05).
HadMSCs were characterized before their expansion to confirm their MSC phenotypes. The results confirmed that the hadMSCs fulfilled the three minimal presentative characteristics of MSCs following the suggestion of The International Society for Cell and Gene Therapy (ISCT2) and in agreement with other studies35, 36. However, the expression of CD105 in our study was higher than that of similar studies (80 — 90%) using different types of culture media such as DMEM—low glucose containing 10% FBS37, 38. This medium expedited a relatively consistent proliferation rate for hadMSCs, expanding from initial (30%) to final confluency (90%; from 0.5 x 106 to 2.5 x 106 cells) after 4 days. Because the cells proliferated and secreted a divergent secretome into a conditioned medium containing animal-free proteins, their secretome profile is safe for therapeutic applications in humans. The results indicated that these experimental cells belonged to high-purity MSCs and were kept fully intact of MSC phenotypes throughout passages 3 to 7 of hadMSC culture in this study39.
Moreover, MSCs have been recognized to exhibit important regenerative properties through the secretion of proangiogenic factors in their exosomes7. In this study, we successfully isolated and characterized exosomes from the conditioned medium of hadMSCs based on the results of their morphology and their expression of specific surface markers. The Bradford assay data relatively measured the total proteins of exosomes, which was 0.51 ± 0.04 µg/mL (n = 4) when expanding the cells for 48 hours.
Furthermore, flow cytometry data showed the percentages of exosome-specific marker expression of CD9, CD63, CD81 were 56.5 ± 6.08%, 87.82 ± 2.29%, and 56.36 ± 16.7%, respectively. Compared to the previous study40, our results exhibited higher expression of exosome markers. A possible reason might come from the ratio of beads versus exosome particles when we increased by 10 times more than the suggested protocol34. Furthermore, the co-expression of the three markers CD9, CD63, and CD81 showed 26.37 ± 5.09%. Moreover, exosome morphology by TEM imaging confirmed the majority of these nanovesicles to be morphologically compatible with defined exosomes. The diameter of the exosomes homogeneously ranged around 150 nm, which was larger than previously observed by other authors, but they were still in the range between 50 and 150 nm35, 41. Therefore, these results reflect the successful isolation and identification of hadMSC-Exo following the suggestion of the International Society for Extracellular Vesicles (MISEV42).
HadMSC-Exo has been previously demonstrated to promote angiogenesis in vitro in HUVEC models43, 25, 44. Previously, many studies have inspected a range of concentrations of hadMSC-Exo from 5 to 100 µg/mL with positive effects on HUVEC models26, 35, 43. Based on our capacity and the amount of exosome obtained in this study, treatment using the concentration of hadMSC-Exo (20 µg/mL) was chosen to examine HUVECs. The results showed that the hadMSC-Exo significantly induced the expression of angiogenesis-related mRNA.
Notably, Han et al. found that the proliferation of HUVECs peaked at 12 hours, implying that the effect of hadMSC-Exo was temporary and expired after 12 hours45. The tracking of the uptake of the fluorescence label hadMSC-Exo demonstrated that after 24 hours, the exosome uptake efficiency was approximately three-fold increased compared to 12 hours43. Therefore, we decided to treat hadMSC-Exo for 24 hours on HUVECs.
Initially, the quiescent phenotype of endothelial cells is modulated by continuous inhibition of growth and enhancement of stabilization signals. Interendothelial junctions serve an important role in the regulation of angiogenesis among adhesive interactions of endothelial cells with each other46. Two vascular cell-specific cell–cell adhesion molecules have been ascertained that localize to endothelial cell intercellular junctions: VE-cadherin and PECAM-1/CD31. The results revealed the significant expression of VE-cadherin by 7.25 ± 2.30 (P < 0.05) and CD31 by 3.02 ± 1.13 (P < 0.05). VE-cadherin is involved in vascular morphogenesis and endothelial survival47. VE-cadherin is a strictly endothelial-specific adhesion molecule located at junctions between endothelial cells. VE-cadherin is vital for the maintenance and control of endothelial cell contacts because it acts as an endothelial switch from the quiescent to the angiogenic phenotype48. Additionally, CD31 plays a critical role in angiogenesis, regulation of cellular responsiveness, and cell survival signals47. During angiogenesis, the endothelial adherens junction is a downstream target of VEGFR-2 signaling that may be involved in the loosening of cell–cell contacts in established vessels to mediate transendothelial permeability and allow sprouting and cell migration46. The expression of Vwf, which is restricted to endothelial cells and the regulation of homeostasis, was not statistically significantly increased, but a trend existed toward increased expression49.
Angiogenesis is a sophisticatedly balanced process, under the control of and fine-tuned by many growth factors. In this study, hadMSC-Exo significantly increased the mRNA expression of Flk-1 or VEGFR-2 in HUVECs by 4.04 ± 0.01 compared to the control group (P < 0.05). This result is similar to a recent study45. This VEGFA/VEGFR2 signaling may act in an autocrine and paracrine fashion in HUVECs to facilitate different signaling pathways in angiogenesis. Moreover, one hypothesis is that during exosome–cytomembrane contact, the angiogenic proteins that were enclosed within the exosome become exposed and begin to exert their effects. The promoting impact of angiogenic proteins might reduce after being endocytosed and degraded by target cells. Nonetheless, further research is required to clarify the mechanism of this phenomenon. On the other hand, the significant mRNA expression of VEGF2 may indicate the presence of the VEGF-A derived from the exosomes, contributing to the uptake and response of HUVECs to this critical angiogenic stimulator. Ordinarily, VEGF substantially represents a growth factor with critical pro-angiogenic signaling pathways that sensitizes endothelial cells to trigger the process of angiogenesis50. This might be reasonable because a fraction of the exosomes might be broken to expose their content during preservation. In addition, RT-qPCR data showed that mRNA expression of VEGFR-1 was dominated by VEGFR-2, a competitive receptor of VEGFR-1 for VEGF (4.04 ± 0.01, P < 0.05). VEGFR-1 has been demonstrated to pose a 10-fold higher affinity than VEGFR-2 but is merely phosphorylated in response to VEGF at low levels51. Specifically, it was demonstrated that when the process was initiated, tip cells were high in VEGFR2, receiving a VEGF-A signal that chemotactically drives migration but does not induce proliferation32. This result was in agreement with Liang et al. (2016) after 48 hours of exosome treatment44.
Regarding different growth factors identified in HUVECs, the mRNA expression of TGFβ appeared to be synthesized remarkably by 11.57 ± 2.03 under the treatment of exosomes (P < 0.05) compared to the control group. TGFβ was shown to exert an antiproliferative property in 2D culture52. In contrast, no difference existed in the expression of Ang1, Ang2, or HGF between the exosome treatment and control groups. From the results, the difference in mRNA expression in the growth factors may imply the spatiotemporal coordination of these growth factors on each other in a specific tendency. In a holistic picture, the angiogenesis-related mRNA expression pattern revealed that the HUVEC was likely to loosen their cell interaction, driving them into the migration stage of angiogenesis under the treatment of exosomes. Moreover, there was no specific stimuli gradient since exosomes were distributed evenly in the in vitro culture, so this could be a possible explanation for the insignificant activation of the expression of angiogenesis-related mRNA such as Ang1, Ang2, HGF, and Vwf. Early during VEGF stimulation, the cellular ratio of Ang2 to Ang1 increases and then decreases at later time points53. The functions of Ang2 appear to be more complex, and Ang2 probably acts as an antagonist of Tie2 signaling to loosen the vessel structure54, whereas Ang1 plays a role in vessel stabilization in the latter stage of angiogenesis. In this 2D culture, tube formation was not formed; therefore, the two genes were not significantly upregulated. In addition, HGF is known as a potent antifibrotic cytokine that prevents tubular epithelial to mesenchymal transition, which was induced by TGF-β55. Meanwhile, TGF-β was remarkably upregulated, and this factor tended to facilitate the migration of HUVECs, so it may act as an antagonist of HGF as the results have demonstrated. In addition, the presence of VEGF may act as a regulator of the switch between migration and proliferation. In the presence of TGFβ signaling, it synergizes with VEGF to navigate migration, while also blocking proliferation51. TGFβ also directly downregulates VEGFR2 transcription56.
During vessel development and maturation, this process is locally controlled and involves tight regulation between growth factors and specific membrane subdomains, such as junctioning, migrationing, and stabilizing, in autocrine and paracrine fashions57. VEGFRs require additional co-receptors to function throughout the process of sprouting angiogenesis. These co-receptors interact either directly or indirectly with the receptor or are associated with VEGFRs in specific membrane subdomains such as the adherens junction58. Specifically, 15 minutes to 1 hour after VEGF stimulation, the endothelial adherens junction components VE-cadherin and PECAM-1/CD31 were maximally phosphorylated on tyrosine. VEGF is believed to transduce a survival signal to endothelial cells through a VE-cadherin-dependent mechanism59. Moreover, VE-cadherin may interfere with VEGFR-2 internalization and reduces its proliferative signal, limiting endothelial cell growth and controlling vascular remodeling46. Furthermore, TGFβ has been demonstrated to modulate the expression of a number of genes in endothelial cells, particularly those involved in the establishment of, and interaction with, the basement51. VE-cadherin may engage in maximal activation of the TGFβ pathway, serving as a positive regulator of TGFβ-induced transcriptional antiproliferative responses in endothelial cells. The increased TGFβ signaling may contribute to VE-cadherin-dependent stabilization and remodeling of the vascular endothelium60. Altogether, these observations are consistent with the high mRNA expression of TGFβ and VE-cadherin of HUVECs in our study.
Conclusions
Our findings suggest that exosomes derived from hadMSCs can promote angiogenesis in vitro. This study further contributes to the understanding of proangiogenic functions and the effects of hadMSC-Exo on endothelial cells via angiogenesis-related mRNA expression. Further attempts should be made to shed light on the clinical application of exosome-based therapies.
Abbreviations
Evs: extracellular vesicles, hadMSC-Exo: exosomes derived from hadMSCs, hadMSCs: human adipose tissue-derived mesenchymal stem cells, HUVECs: Human umbilical vein endothelial cells, MSCs: mesenchymal stem cells
Acknowledgments
We thank SCI Biobank for providing mesenchymal stem cells used in this study.
Author’s contributions
Xuan-Quynh Tran and Bich-Ngoc Vu equally contributed to this work. All authors read and approved the final version of the manuscript.
Funding
This research was funded by Vietnam National University, Ho Chi Minh City (VNU-HCM) under grant number 562-2020-18-03.
Availability of data and materials
Data and materials used and/or analyzed during the current study are available from the corresponding author on reasonable request.
Ethics approval and consent to participate
Not applicable.
Consent for publication
Not applicable.
Competing interests
The authors declare that they have no competing interests.
References
-
Pittenger
M.F.,
Discher
D.E.,
Péault
B.M.,
Phinney
D.G.,
Hare
J.M.,
Caplan
A.I.,
Mesenchymal stem cell perspective: cell biology to clinical progress. NPJ Regenerative Medicine.
2019;
4
(1)
:
22
.
View Article PubMed Google Scholar -
Viswanathan
S.,
Shi
Y.,
Galipeau
J.,
Krampera
M.,
Leblanc
K.,
Martin
I.,
Mesenchymal stem versus stromal cells: International Society for Cell & Gene Therapy (ISCT) Mesenchymal Stromal Cell committee position statement on nomenclature. Cytotherapy.
2019;
21
(10)
:
1019-24
.
View Article PubMed Google Scholar -
Maqsood
M.,
Kang
M.,
Wu
X.,
Chen
J.,
Teng
L.,
Qiu
L.,
Adult mesenchymal stem cells and their exosomes: Sources, characteristics, and application in regenerative medicine. Life Sciences.
2020;
256
:
118002
.
View Article PubMed Google Scholar -
Guo
Y.,
Yu
Y.,
Hu
S.,
Chen
Y.,
Shen
Z.,
The therapeutic potential of mesenchymal stem cells for cardiovascular diseases. Cell Death & Disease.
2020;
11
(5)
:
349
.
View Article PubMed Google Scholar -
Bunnell
B.A.,
Flaat
M.,
Gagliardi
C.,
Patel
B.,
Ripoll
C.,
Adipose-derived stem cells: isolation, expansion and differentiation. Methods (San Diego, Calif.).
2008;
45
(2)
:
115-20
.
View Article PubMed Google Scholar -
Mathivanan
S.,
Ji
H.,
Simpson
R.J.,
Exosomes: extracellular organelles important in intercellular communication. Journal of Proteomics.
2010;
73
(10)
:
1907-20
.
View Article PubMed Google Scholar -
Alcayaga-Miranda
F.,
Varas-Godoy
M.,
Khoury
M.,
Harnessing the Angiogenic Potential of Stem Cell-Derived Exosomes for Vascular Regeneration. Stem Cells International.
2016;
2016
:
3409169
.
View Article PubMed Google Scholar -
Baglio
S.R.,
Rooijers
K.,
Koppers-Lalic
D.,
Verweij
F.J.,
Lanzón
M. Pérez,
Zini
N.,
Human bone marrow- and adipose-mesenchymal stem cells secrete exosomes enriched in distinctive miRNA and tRNA species. Stem Cell Research and Therapy.
2015;
6
(1)
:
127
.
View Article PubMed Google Scholar -
Xu
C.,
Lin
L.,
Cao
G.,
Chen
Q.,
Shou
P.,
Huang
Y.,
Interferon-α-secreting mesenchymal stem cells exert potent antitumor effect in vivo. Oncogene.
2014;
33
(42)
:
5047-52
.
View Article PubMed Google Scholar -
Rezakhani
L.,
Kelishadrokhi
A.F.,
Soleimanizadeh
A.,
Rahmati
S.,
Mesenchymal stem cell (MSC)-derived exosomes as a cell-free therapy for patients Infected with COVID-19: real opportunities and range of promises. Chemistry and Physics of Lipids.
2021;
234
:
105009
.
View Article PubMed Google Scholar -
Liang
Y.,
Duan
L.,
Lu
J.,
Xia
J.,
Engineering exosomes for targeted drug delivery. Theranostics.
2021;
11
(7)
:
3183-95
.
View Article PubMed Google Scholar -
Wang
Y.,
Zhang
Z.,
Chi
Y.,
Zhang
Q.,
Xu
F.,
Yang
Z.,
Long-term cultured mesenchymal stem cells frequently develop genomic mutations but do not undergo malignant transformation. Cell Death & Disease.
2013;
4
(12)
:
e950-950
.
View Article PubMed Google Scholar -
Ullah
M.,
Stich
S.,
Notter
M.,
Eucker
J.,
Sittinger
M.,
Ringe
J.,
Transdifferentiation of mesenchymal stem cells-derived adipogenic-differentiated cells into osteogenic- or chondrogenic-differentiated cells proceeds via dedifferentiation and have a correlation with cell cycle arresting and driving genes. Differentiation.
2013;
85
(3)
:
78-90
.
View Article PubMed Google Scholar -
Mohseny
A.B.,
Szuhai
K.,
Romeo
S.,
Buddingh
E.P.,
Briaire-de Bruijn
I.,
de Jong
D.,
Osteosarcoma originates from mesenchymal stem cells in consequence of aneuploidization and genomic loss of Cdkn2. The Journal of Pathology.
2009;
219
(3)
:
294-305
.
View Article PubMed Google Scholar -
Askar
S.F.,
Ramkisoensing
A.A.,
Atsma
D.E.,
Schalij
M.J.,
de Vries
A.A.,
Pijnappels
D.A.,
Engraftment patterns of human adult mesenchymal stem cells expose electrotonic and paracrine proarrhythmic mechanisms in myocardial cell cultures. Circulation: Arrhythmia and Electrophysiology.
2013;
6
(2)
:
380-91
.
View Article PubMed Google Scholar -
Vizoso
F.J.,
Eiro
N.,
Cid
S.,
Schneider
J.,
Perez-Fernandez
R.,
Mesenchymal Stem Cell Secretome: Toward Cell-Free Therapeutic Strategies in Regenerative Medicine. International Journal of Molecular Sciences.
2017;
18
(9)
:
1852
.
View Article PubMed Google Scholar -
Bermudez
M.A.,
Sendon-Lago
J.,
Seoane
S.,
Eiro
N.,
Gonzalez
F.,
Saa
J.,
Anti-inflammatory effect of conditioned medium from human uterine cervical stem cells in uveitis. Experimental Eye Research.
2016;
149
:
84-92
.
View Article PubMed Google Scholar -
Fang
Y.,
Zhang
Y.,
Zhou
J.,
Cao
K.,
Adipose-derived mesenchymal stem cell exosomes: a novel pathway for tissues repair. Cell and Tissue Banking.
2019;
20
(2)
:
153-61
.
View Article PubMed Google Scholar -
Maria
A.T.,
Maumus
M.,
Quellec
A. Le,
Jorgensen
C.,
Noël
D.,
Guilpain
P.,
Adipose-Derived Mesenchymal Stem Cells in Autoimmune Disorders: State of the Art and Perspectives for Systemic Sclerosis. Clinical Reviews in Allergy & Immunology.
2017;
52
(2)
:
234-59
.
View Article PubMed Google Scholar -
Venkatesh
K.,
Sen
D.,
Mesenchymal Stem Cells as a Source of Dopaminergic Neurons: A Potential Cell Based Therapy for Parkinson's Disease. Current Stem Cell Research and Therapy.
2017;
12
(4)
:
326-47
.
View Article PubMed Google Scholar -
Trzil
J.E.,
Masseau
I.,
Webb
T.L.,
Chang
C.H.,
Dodam
J.R.,
Liu
H.,
Intravenous adipose-derived mesenchymal stem cell therapy for the treatment of feline asthma: a pilot study. Journal of Feline Medicine and Surgery.
2016;
18
(12)
:
981-90
.
View Article PubMed Google Scholar -
Baharlou
R.,
Rashidi
N.,
Ahmadi-Vasmehjani
A.,
Khoubyari
M.,
Sheikh
M.,
Erfanian
S.,
Immunomodulatory Effects of Human Adipose Tissue-derived Mesenchymal Stem Cells on T Cell Subsets in Patients with Rheumatoid Arthritis. Iranian Journal of Allergy, Asthma, and Immunology.
2019;
18
(1)
:
114-9
.
View Article PubMed Google Scholar -
Wang
Z.G.,
He
Z.Y.,
Liang
S.,
Yang
Q.,
Cheng
P.,
Chen
A.M.,
Comprehensive proteomic analysis of exosomes derived from human bone marrow, adipose tissue, and umbilical cord mesenchymal stem cells. Stem Cell Research and Therapy.
2020;
11
(1)
:
511
.
View Article PubMed Google Scholar -
Luo
Q.,
Guo
D.,
Liu
G.,
Chen
G.,
Hang
M.,
Jin
M.,
Exosomes from MiR-126-Overexpressing Adscs Are Therapeutic in Relieving Acute Myocardial Ischaemic Injury. Cellular Physiology and Biochemistry.
2017;
44
(6)
:
2105-16
.
View Article PubMed Google Scholar -
Shabbir
A.,
Cox
A.,
Rodriguez-Menocal
L.,
Salgado
M.,
Van Badiavas
E.,
Mesenchymal Stem Cell Exosomes Induce Proliferation and Migration of Normal and Chronic Wound Fibroblasts, and Enhance Angiogenesis In Vitro. Stem Cells and Development.
2015;
24
(14)
:
1635-47
.
View Article PubMed Google Scholar -
Bian
S.,
Zhang
L.,
Duan
L.,
Wang
X.,
Min
Y.,
Yu
H.,
Extracellular vesicles derived from human bone marrow mesenchymal stem cells promote angiogenesis in a rat myocardial infarction model. Journal of Molecular Medicine (Berlin, Germany).
2014;
92
(4)
:
387-97
.
View Article PubMed Google Scholar -
Xu
H.,
Wang
Z.,
Liu
L.,
Zhang
B.,
Li
B.,
Exosomes derived from adipose tissue, bone marrow, and umbilical cord blood for cardioprotection after myocardial infarction. Journal of Cellular Biochemistry.
2020;
121
(3)
:
2089-102
.
View Article PubMed Google Scholar -
Zhang
D.,
Lee
H.,
Zhu
Z.,
Minhas
J.K.,
Jin
Y.,
Enrichment of selective miRNAs in exosomes and delivery of exosomal miRNAs in vitro and in vivo. American Journal of Physiology. Lung Cellular and Molecular Physiology.
2017;
312
(1)
:
110-21
.
View Article PubMed Google Scholar -
Ni
J.,
Li
H.,
Zhou
Y.,
Gu
B.,
Xu
Y.,
Fu
Q.,
Therapeutic Potential of Human Adipose-Derived Stem Cell Exosomes in Stress Urinary Incontinence - An in Vitro and in Vivo Study. Cellular Physiology and Biochemistry.
2018;
48
(4)
:
1710-22
.
View Article PubMed Google Scholar -
Anderson
J.D.,
Johansson
H.J.,
Graham
C.S.,
Vesterlund
M.,
Pham
M.T.,
Bramlett
C.S.,
Comprehensive Proteomic Analysis of Mesenchymal Stem Cell Exosomes Reveals Modulation of Angiogenesis via Nuclear Factor-KappaB Signaling. Stem Cells (Dayton, Ohio).
2016;
34
(3)
:
601-13
.
View Article PubMed Google Scholar -
Hoang
D.H.,
Nguyen
T.D.,
Nguyen
H.P.,
Nguyen
X.H.,
Do
P.T.,
Dang
V.D.,
Differential Wound Healing Capacity of Mesenchymal Stem Cell-Derived Exosomes Originated From Bone Marrow, Adipose Tissue and Umbilical Cord Under Serum- and Xeno-Free Condition. Frontiers in Molecular Biosciences.
2020;
7
:
119
.
View Article PubMed Google Scholar -
Gerhardt
H.,
Betsholtz
C.,
Endothelial-pericyte interactions in angiogenesis. Cell and Tissue Research.
2003;
314
(1)
:
15-23
.
View Article PubMed Google Scholar -
Kuehbacher
A.,
Urbich
C.,
Dimmeler
S.,
Targeting microRNA expression to regulate angiogenesis. Trends in Pharmacological Sciences.
2008;
29
(1)
:
12-5
.
View Article PubMed Google Scholar -
Théry
C.,
Amigorena
S.,
Raposo
G.,
Clayton
A.,
Isolation and characterization of exosomes from cell culture supernatants and biological fluids. Current Protocols in Cell Biology.
2006;
Chapter 3
(1)
:
22
.
View Article PubMed Google Scholar -
Heo
J.S.,
Kim
S.,
Human adipose mesenchymal stem cells modulate inflammation and angiogenesis through exosomes. Scientific Reports.
2022;
12
(1)
:
2776
.
View Article PubMed Google Scholar -
Kalinina
N.,
Kharlampieva
D.,
Loguinova
M.,
Butenko
I.,
Pobeguts
O.,
Efimenko
A.,
Characterization of secretomes provides evidence for adipose-derived mesenchymal stromal cells subtypes. Stem Cell Research and Therapy.
2015;
6
(1)
:
221
.
View Article PubMed Google Scholar -
Heo
J.S.,
Kim
S.,
Yang
C.E.,
Choi
Y.,
Song
S.Y.,
Kim
H.O.,
Human Adipose Mesenchymal Stem Cell-Derived Exosomes: A Key Player in Wound Healing. Tissue Engineering and Regenerative Medicine.
2021;
18
(4)
:
537-48
.
View Article PubMed Google Scholar -
Lin
R.,
Wang
S.,
Zhao
R.C.,
Exosomes from human adipose-derived mesenchymal stem cells promote migration through Wnt signaling pathway in a breast cancer cell model. Molecular and Cellular Biochemistry.
2013;
383
(1-2)
:
13-20
.
View Article PubMed Google Scholar -
Truong
N.C.,
Bui
K.H.,
Pham
P. Van,
Characterization of Senescence of Human Adipose-Derived Stem Cells After Long-Term Expansion. Adv Exp Med Biol.
2019;
1084
:
109-128
.
View Article PubMed Google Scholar -
González-Cubero
E.,
González-Fernández
M.L.,
Gutiérrez-Velasco
L.,
Navarro-Ramírez
E.,
Villar-Suárez
V.,
Isolation and characterization of exosomes from adipose tissue-derived mesenchymal stem cells. Journal of Anatomy.
2021;
238
(5)
:
1203-17
.
View Article PubMed Google Scholar -
Xiao
S.,
Xiao
C.,
Miao
Y.,
Wang
J.,
Chen
R.,
Fan
Z.,
Human acellular amniotic membrane incorporating exosomes from adipose-derived mesenchymal stem cells promotes diabetic wound healing. Stem Cell Research & Therapy.
2021;
12
(1)
:
255
.
View Article PubMed Google Scholar -
Théry
C.,
Witwer
K.W.,
Aikawa
E.,
Alcaraz
M.J.,
Anderson
J.D.,
Andriantsitohaina
R.,
Minimal information for studies of extracellular vesicles 2018 (MISEV2018): a position statement of the International Society for Extracellular Vesicles and update of the MISEV2014 guidelines. Journal of Extracellular Vesicles.
2018;
7
(1)
:
1535750
.
View Article PubMed Google Scholar -
Xue
C.,
Shen
Y.,
Li
X.,
Li
B.,
Zhao
S.,
Gu
J.,
Exosomes Derived from Hypoxia-Treated Human Adipose Mesenchymal Stem Cells Enhance Angiogenesis Through the PKA Signaling Pathway. Stem Cells and Development.
2018;
27
(7)
:
456-65
.
View Article PubMed Google Scholar -
Liang
X.,
Zhang
L.,
Wang
S.,
Han
Q.,
Zhao
R.C.,
Exosomes secreted by mesenchymal stem cells promote endothelial cell angiogenesis by transferring miR-125a. Journal of Cell Science.
2016;
129
(11)
:
2182-9
.
View Article PubMed Google Scholar -
Han
Y.,
Ren
J.,
Bai
Y.,
Pei
X.,
Han
Y.,
Exosomes from hypoxia-treated human adipose-derived mesenchymal stem cells enhance angiogenesis through VEGF/VEGF-R. The International Journal of Biochemistry & Cell Biology.
2019;
109
:
59-68
.
View Article PubMed Google Scholar -
Esser
S.,
Lampugnani
M.G.,
Corada
M.,
Dejana
E.,
Risau
W.,
Vascular endothelial growth factor induces VE-cadherin tyrosine phosphorylation in endothelial cells. Journal of Cell Science.
1998;
111
(Pt 13)
:
1853-65
.
View Article PubMed Google Scholar -
Wallez
Y.,
Vilgrain
I.,
Huber
P.,
Angiogenesis: the VE-cadherin switch. Trends in Cardiovascular Medicine.
2006;
16
(2)
:
55-9
.
View Article PubMed Google Scholar -
Li
S.Q.,
Lin
Y.N.,
Li
Q.Y.,
Soluble VE-cadherin: not just a marker of endothelial permeability. The European Respiratory Journal.
2021;
58
(6)
:
2102241
.
View Article PubMed Google Scholar -
Mojzisch
A.,
Brehm
M.A.,
The Manifold Cellular Functions of von Willebrand Factor. Cells.
2021;
10
(9)
:
2351
.
View Article PubMed Google Scholar -
Melincovici
C.S.,
Boşca
A.B.,
\cSuşman
S.,
M\uarginean
M.,
Mihu
C.,
Istrate
M.,
Vascular endothelial growth factor (VEGF) - key factor in normal and pathological angiogenesis. Romanian Journal of Morphology and Embryology.
2018;
59
(2)
:
455-67
.
PubMed Google Scholar -
Holderfield
M.T.,
Hughes
C.C.,
Crosstalk between vascular endothelial growth factor, notch, and transforming growth factor-β in vascular morphogenesis. Circulation Research.
2008;
102
(6)
:
637-52
.
View Article PubMed Google Scholar -
Pepper
M.S.,
Transforming growth factor-beta: vasculogenesis, angiogenesis, and vessel wall integrity. Cytokine & Growth Factor Reviews.
1997;
8
(1)
:
21-43
.
View Article PubMed Google Scholar -
Singh
H.,
Hansen
T.M.,
Patel
N.,
Brindle
N.P.,
The molecular balance between receptor tyrosine kinases Tie1 and Tie2 is dynamically controlled by VEGF and TNFα and regulates angiopoietin signalling. PLoS One.
2012;
7
(1)
:
e29319
.
View Article PubMed Google Scholar -
Maisonpierre
P. C.,
Suri
C.,
Jones
P. F.,
Bartunkova
S.,
Wiegand
S. J.,
Radziejewski
C.,
Compton
D.,
McClain
J.,
Aldrich
T. H.,
Papadopoulos
N.,
Angiopoietin-2, a natural antagonist for Tie2 that disrupts in vivo angiogenesis. Science.
1997;
277
(5322)
:
55-60
.
View Article PubMed Google Scholar -
Yang
J.,
Dai
C.,
Liu
Y.,
A novel mechanism by which hepatocyte growth factor blocks tubular epithelial to mesenchymal transition. Journal of the American Society of Nephrology.
2005;
16
(1)
:
68-78
.
View Article PubMed Google Scholar -
Minami
T.,
Rosenberg
R.D.,
Aird
W.C.,
Transforming growth factor-β 1-mediated inhibition of the flk-1/KDR gene is mediated by a 5'-untranslated region palindromic GATA site. The Journal of Biological Chemistry.
2001;
276
(7)
:
5395-402
.
View Article PubMed Google Scholar -
Ramsauer
M.,
D'Amore
P.A.,
Contextual role for angiopoietins and TGFbeta1 in blood vessel stabilization. Journal of Cell Science.
2007;
120
(Pt 10)
:
1810-7
.
View Article PubMed Google Scholar -
Joussen
A.M.,
Kirchhof
B.,
Gottstein
C.,
[Molecular mechanisms of vasculogenesis and angiogenesis. What regulates vascular growth?]. Der Ophthalmologe.
2003;
100
(4)
:
284-91
.
View Article PubMed Google Scholar -
Koch
S.,
Claesson-Welsh
L.,
Signal transduction by vascular endothelial growth factor receptors. Cold Spring Harbor Perspectives in Medicine.
2012;
2
(7)
:
a006502
.
View Article PubMed Google Scholar -
Rudini
N.,
Felici
A.,
Giampietro
C.,
Lampugnani
M.,
Corada
M.,
Swirsding
K.,
VE-cadherin is a critical endothelial regulator of TGF-beta signalling. The EMBO Journal.
2008;
27
(7)
:
993-1004
.
View Article PubMed Google Scholar
Comments
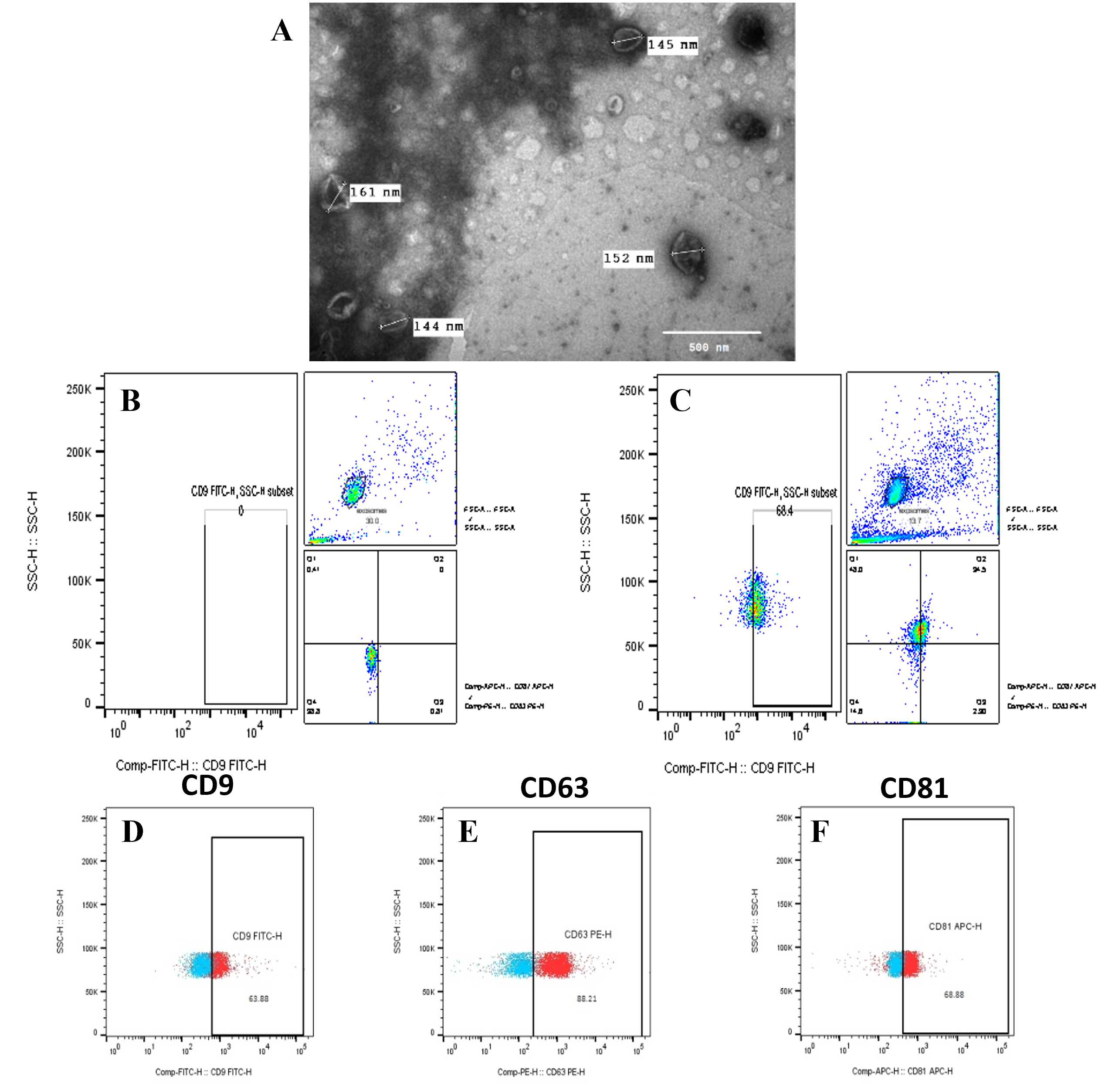
Article Details
Volume & Issue : Vol 9 No 9 (2022)
Page No.: 5278-5290
Published on: 2022-09-30
Citations
Copyrights & License

This work is licensed under a Creative Commons Attribution 4.0 International License.
Search Panel
- HTML viewed - 4496 times
- PDF downloaded - 909 times
- XML downloaded - 0 times