Hepatocyte growth factor improves direct reprogramming of fibroblasts towards endothelial progenitor cells via ETV2 transduction
Abstract
Human fibroblasts can be differentiated into endothelial progenitor cells by direct reprogramming via ETV-2 transfection. Previously, we have shown that the efficacy of direct reprogramming can be enhanced by hypoxia treatment. In this study, we aim to investigate whether the efficacy of direct reprogramming of fibroblasts into EPCs via Ets variant gene 2 (ETV2) transfection can be increased with hepatocyte growth factor (HGF) treatment. Foreskin-derived fibroblasts were cultured in standard medium (DMEM/F12 supplemented with fetal bovine serum). They were then transduced with a viral vector expressing ETV2 in culture medium supplemented with HGF. The transduced fibroblasts were cultured in endothelial cell medium supplemented with HGF for 28 days. The efficacy of direct reprogramming was evaluated based on expression of CD31 and VEGFR2 markers by transduced cells. Phenotypic and functional characterization of induced EPCs were also confirmed by expression of particular genes and in vitro angiogenesis assays. Our results showed that HGF significantly increased the efficacy of direct reprogramming of fibroblasts towards EPCs via ETV2 transcription factors; efficiency increased from 5.41±1.51% for ETV2 transduction alone to 12.31±2.15% for ETV2 transduction combined with HGF treatment. These findings suggest the rationale for combined use of ETV2 and HGF in direct in vitro reprogramming of fibroblasts into EPCs.
Introduction
Endothelial progenitor cells (EPCs) are an important cell source for regenerative medicine Kalka et al., 2000Kondo et al., 2003 as well as tissue engineering Urbich and Dimmeler, 2004. Although EPCs can be isolated from several sources (e.g. bone marrow Asahara et al., 1999Takahashi et al., 1999, peripheral blood, umbilical cord blood and adipose tissue), the percentage of EPCs obtained from these sources are extremely low. Therefore, it is important to have another source or strategy to yield a greater quantity of EPCs.
One strategy to yield EPCs involves the in vitro differentiation of pluripotent stem cells, such as embryonic stem cells (ESCs) and induced pluripotent stem cells (PSCs), into EPCs. Previous studies have shown that it is feasible to differentiate ESCs into EPCs Ferreira et al., 2007Levenberg et al., 2002Vittet et al., 1996Wang et al., 2007. The differentiated cells are capable of forming capillary-like structures in vitro as well as exhibiting therapeutic effects in animals Hirashima et al., 1999Wang et al., 2007. However, ESCs have faced some ethical issues with regard to isolation procedures. Therefore, less focus has been placed on differentiation of ESCs into EPCs; in lieu of that, there has been greater interest in differentiation of induced PSCs into EPCs. Notably, induced PSCs are pluripotent stem cells which are produced by reprogramming of the genome with certain factors. The first publication about induced PSCs was reported by Yamanaka et al. in 2006 Takahashi and Yamanaka, 2006. The authors showed that induced PSCs were produced by skin fibroblasts after transduction with 4 factors: Oct-3/4, cMyc, Klf4 and Sox-2. The induced PSCs were also successfully differentiated into EPCs Choi et al., 2009. However, the biggest limitation related to use of differentiated EPCs from induced PSCs or ESCs has been tumor formation from residual ESCs or induced PSCs inside the differentiated cells. As a way to overcome the limitation of in vitro differentiation of PSCs into EPCs, direct reprogramming strategies have now garnered greater consideration.
The first effort towards direct reprogramming of fibroblasts to EPCs was performed by Margariti et al., 2012. They developed a method to generate partial-induced PSCs by transferring four reprogramming factors (OCT4, SOX2, KLF4, and c-MYC) to human fibroblasts (HFs) for 4 days. These partial-induced PSCs were capable of differentiating into endothelial cells in response to defined media and culture conditions Margariti et al., 2012. In the defined solutions, endothelial cells could not form tumors. However, these EPCs also expressed oncogenes, such as Sox-2. To resolve this, in subsequent studies by Li etal. and Han et al, the Sox- 2 gene was removed using a revised version of the procedure from Margariti et al. Moreover, the authors used two genes (Oct4 and Klf4) in combination with soluble factors Li et al., 2013 or Foxo1, Er71, Klf2, Tal1 and Lmo2 Han et al., 2014.
There are have been limitations to the initial studies mentioned above. Firstly, the authors used many factors to convert fibroblasts into EPCs, making the procedure more complicated. Secondly, the efficacy of the procedure was very low. To conduct the direct reprogramming procedure, the Ets variant gene 2 (ETV2) was recently used as a single factor to induce direct reprogramming of fibroblasts into EPCs Lee et al., 2014Morita et al., 2015. ETV2 is a master gene that regulates various signaling pathways and functions and is an essential regulator of vasculogenesis and hematopoiesis Shi et al., 2014, cardiac development Schupp et al., 2014 and vascular regeneration Park et al., 2016. However, direct reprogramming via ETV2 transduction has been low (about 1 %) Morita et al., 2015. We have tried to improve this efficacy by treatment with hypoxia Van Pham et al., 2016. Using the hypoxia approach, the efficacy of direct reprogramming of fibroblasts into EPCs by single vector ETV2 increased to 3-4% Van Pham et al., 2016. Although the efficacy of the procedure was significantly increased, the percentage of reprogrammed EPCs was still low. Therefore, in this study, we aimed to improve the efficacy of epigenetic reprogramming of fibroblasts into EPCs using single vector ETV2 in combination with hepatocyte growth factor (HGF) treatment.
Materials-methods
Isolation and culture of fibroblasts from foreskin
Human foreskin was collected at hospitals from donors after consent. At the laboratory, foreskin was washed twice with PBS, cut into small fragments and cultured in DMEM/F12 supplemented with 10% FBS at 37°C, 5% CO2 condition. After 5 days, the culture was observed to evaluate the migration of fibroblasts from the fragment. After 20 days, the cultures were sub-cultured to promote fibroblast proliferation. The fibroblasts at the 3rd passage were assessed for expression of CD90 and vimentin by flow cytometry and were used for subsequent experiments. Human umbilical vein endothelial cells (HUVECs) were purchased from Life Technologies, Inc. (code number C0035C; Carlsbad, CA).
Production of ETV2-expressing viral vector
Production of the ETV2-expressing viral vector was performed as described in previous studies Van Pham et al., 2016. Briefly, the human ETV2 expression vector (pF1KB9707) was purchased from Addgene, Inc. (Cambridge, MA). ETV2 was cloned into the vector backbone pSIN4-EF1alpha-IRES-Puro (Plasmid #61061; Addgene, Inc.) to generate pSIN4-EF1a-ETV2-IRES-Puro. All of the coding sequences in the expression vector were confirmed using a GenomeLab System (Beckman Coulter, Brea, CA, USA). The ETV2 vector was then transfected into HEK293T cells along with pCMV-VSV-G-RSV-Rev and pCMV-dR8.2 (Addgene). Eighteen hours after transfection, the medium was replaced with fresh culture medium, and 48 h later the lentivirus-containing medium was collected, passed through a 0.45-μm filter and concentrated using centrifugation (8400 × g at 4 °C for 16 h). The lentivirus pellets were resuspended in PBS at 107 IFUs/mL.
Transduction of ETV2 to fibroblasts
Three 12-well plates were seeded with fibroblasts at 7 x 104 cells per well. The plates were divided into 3 groups: 1) “control” (cells not transduced with viral vector), 2) “ETV2” (cells transduced with ETV2 viral vectors), and 3) “ETV2+HGF” (cells transduced with ETV2 viral vector combined with 10 ng/mL HGF in culture medium). After 24 h of incubation, 10 μL concentrated lentivirus particles with 5 μg/mL protamine were added into each well of the ETV2 and ETV2+HGF groups. Another 48 h later, cells were washed twice with PBS and then cultured in 6-cm dishes coated with Cellstart (Life Technologies, Inc.). Cells in the ETV2 group were plated in EGM-2 medium and cells in the ETV2+HGF group was plated in EGM-2 medium supplemented with 10 ng/mL HGF (Life Technologies).
Flow cytometry and cell sorting
To evaluate the percentage of CD31+VEGFR2+ cells in the 3 groups (control, ETV2 and ETV2+HGF), cells were collected after 14 days and 28 days by trypsinization with trypsin/EDTA (0.04% EDTA). The cells were resuspended in PBS containing 2 % FBS and 2 mM EDTA, and then stained with fluorochromeleveled mAbs for 20 min on ice. Two kinds of mAbs were used: anti-human CD31-FITC and anti-human VEGFR2-PE (BD Biosciences, San Jose, C). The expression of these markers were analyzed by a FACSCalibur flow cytometer (BD Biosciences). At day 28, after analysis the CD31+VEGFR2+ cells in the ETV2 and ETV2+HGF groups were sorted using a FACSJazz cell sorter (BD Biosciences). These CD31+VEGFR2+ cells were used for further assays.
Real-time RT-PCR
The sorted CD31+VEGFR2+ cells were used in real-time reverse transcriptase (RT)-PCR. Total RNA was extracted using Trizol according to the manufacturer’s instructions. PCR analysis was performed using onestep real-time RT-PCR and the SYBR® Green Quantitative RT-qPCR Kit (Sigma-Aldrich, St. Louis, MO) on a Realplex Mastercycler (Eppendorf, Hamburg, Germany). Relative gene expression levels were normalized by comparison to GAPDH. Genespecific primer pairs are presented in Table 1 .
Capillary-like structure formation assay
The sorted CD31+VEGFR2+ cells were used in capillary-like formation assay. Cells from the ETV2 and ETV2+HGF groups at day 28 were seeded on 96-well flat-bottom plates coated with 30 μL Matrigel (Life Technologies) and cultured in EGM-2 medium at 2 × 104 cells/well. Eighteen hours after incubation, capillary-like structures were observed under an Axiovert microscope (Carl-Zeiss, Oberkochen, Germany).
Statistical analysis
Statistical analyses of all endpoints were performed using the two-sided Student’s t test or one-way analysis of variance. All data are presented as mean ± SD. p < 0.05 was considered statistically significant. Data were analyzed with GraphPad Prism 6.0 software.
Results
Culture and characterization of foreskin-derived fibroblasts
The fibroblasts started to migrate from the fragments at day 5. The cells proliferated over 21 days and the cell population was continuously sub-cultured until the 3rd passage to yield a homogenous cell population ( Figure 1A ). Fibroblast phenotype was confirmed by expression of CD90 ( Figure 1B ) and vimentin ( Figure 1C ). The results showed that 100% cells expressed both CD90 and vimentin.
Expression of CD31 and VEGFR2 in transduced fibroblasts
The sub-population with the CD31+VEGFR2+ phenotype was identified by flow cytometry. The results are represented in Figure 2 . After 14 days, the percentage of the CD31+VEGFR2+ cell population was 4.01±2.12%, 2.81±1.51%, and 0% in the control, ETV2 and ETV2+HGF groups, respectively ( Figure 2A ). There was no significantly difference between ETV2 and ETV2+HGF groups. However, after 28 days there was a significant difference in the CD31+VEGFR2+ population of the control, ETV2 and ETV2+HGF groups; the percentage was 12.31±2.15%, 5.41±1.51%, and 0% for control, ETV2 and ETV2+HGF groups, respectively ( Figure 2B ).
Profile of gene expression related to EPCs
The CD31+VEGFR2+ cells were sorted from transduced cells of the ETV2 or ETV2-HGF groups. The purified cells were evaluated for expression of NRP2, NR2F2, EPHB4, JAG1, EFNB2, HEY1, PROX2, and SOX18(all these genes are expressed in induced EPCs). The results showed that expression of these genes significantly increased in both ETV2 and ETV2+HGF groups, compared to the non-transduced (control) group. However, the expression of these genes were lower than that typically expressed by HUVECs. Although the expression of these genes were different between the ETV2 and ETV2+HGF 2 groups, the differences were non-significant after normalization to GAPDH (p > 0.05), except for the EFNB2 gene ( Figure 3 ).
The capillary like structure formation of induced EPCs
To evaluate the biological activities of induced EPCs in some groups, the sorted CD31+VEGFR2+ cells were cultured in matrigel to determine the capillary-like structure formation. After 18 h of incubation, CD31+VEGFR2+ cells from both the ETV2 and ETV2+HGF groups could form capillary-like structures similar to HUVECs ( Figure 4 ). However, the fibroblasts could not form these structures.
Discussion
EPCs are an important cell source for regenerative medicine and tissue engineering. EPCs can be used to treat ischemia-related diseases as well as to produce blood vessels. However, the source of EPCs in some tissues are so low and very difficult to culture and proliferate in vitro. This study provided a new method to efficiently produce EPCs in vitro for applications through direct reprogramming of fibroblasts.
In a previous study, Morita et al. (2014) demonstrated success in directly converting fibroblasts into EPCs using a single gene factor (ETV2). Recently, the efficacy of this procedure was improved upon with hypoxia treatment. Using a hypoxic condition, the efficacy of the procedure significantly increased from 3% to 7% Van Pham et al., 2016. In the study herein, we improved the procedure further via treatment of fibroblasts with HGF during the converting procedure.
This study also confirms the roles of HGF in endothelial differentiation and reprogramming. The reprogrammed cells under HGF treatment had same similar properties as the control. They exhibited not only EPC phenotype but also in vitro function. They expressed the particular markers of EPCs (e.g. CD31 and VEGFR2). CD31 and VEGFR2 are two popular markers of EPCs which have been used to identify and confirm EPC populations in several previous studies. These markers are also expressed in EPCs derived from bone marrow, peripheral blood and umbilical cord blood Amini et al., 2012Cherqui et al., 2006Hristov et al., 2003Masek and Sweetenham, 1994Mead et al., 2008. The reprogrammed cells could also exhibit capillary-like structure formation in vitro Mead et al., 2008. Overall, in the conditions with ETV2 transduction alone or ETV2+HGF, induced EPCs exhibited the functional EPC phenotypes.
However, quantitative analysis showed that the percentage of the CD31+VEGFR2+ cell population in the ETV2+HGF group was significant higher than that of the ETV2 group. Our results confirm that HGF supplement in the endothelial cell culture medium increases the percentage of induced EPCs. The mechanism of HGF effects on the direct reprogramming of fibroblasts toward induced EPCs via ETV2 was not investigated in this study. However, based on some previous studies examining the roles of HGF, there are some suggestions as to the mechanism of HGF in our procedure.
HGF efficiently stimulates the proliferation of induced EPCs. With this mechanism, the initial direct reprogramming in the ETV2 and ETV2+HGF groups could be similar. However, after 21 days under HGF treatment condition, HGF may stimulate proliferation of induced EPCs. Thus, at day 21 the percentage of induced EPCs in the ETV2+HGF group was higher than that of the HGF group and that of the ETV2 group. Indeed, Song et al. 2009 transfected HGF into EPCs and showed that transfection of EPCs with HGFenhanced the proliferative, migratory and angiogenic capabilities, and promoted the activation of ERK1/2 without affecting its expression. Interestingly, in the study of Zhu et al. 2010, the authors determined that HGF secreted from smooth muscle cells played a role in the regulation of EPC differentiation, migration and proliferation. They also showed that exogenous recombinant HGF markedly up-regulated eNOS mRNA expression in EPCs and promoted EPC migration and proliferation, while bearing no significant changes in KDR and CD31 mRNA expression Zhu et al., 2010.
In another study, HGF was shown to be the factor responsible for inducing the pro-angiogenic phenotype and mobilizing EPCs by activating Nox2 Schroder et al., 2011. Furthermore, HGF protected EPCs from damage of low-density lipoprotein cholesterol via the PI3K/Akt signaling pathway Yu et al., 2010, prevented advanced glycation end productinduced injury Zhou et al., 2006, prevented oxidative stress through a PI3K/Akt-dependent pathway Zhou et al., 2009, and attenuated angiotensin II-induced endothelial progenitor cell senescence Sanada et al., 2009. HGF in vivo can induce angiogenesis through mobilizing EPCs Ishizawa et al., 2004. Collectively, the effects of HGF on improving direct reprogramming of fibroblasts into EPCs by ETV2 may be an indirect one. This may mean that HGF does not participate in any signaling pathways to change the epigenetics which help fibroblasts acquire the EPC phenotype, but that HGF can stimulate the proliferation and maturation of the reprogrammed EPCs.
Conclusion
EPCs have been widely used in stem cell therapy and tissue engineering. Our study herein describes an enhancement of direct reprogramming of fibroblasts into induced EPCs by single viral vector of ETV2 in combination with HGF. The induced cells exhibited the functional phenotype of EPCs, including CD31 and VEGFR2 expression, gene profile related to EPCs, and capillary-like structure formation. The role of HGF may be to directly affect the reprogramming signaling pathway or indirectly stimulate proliferation of induced cells. Our findings demonstrate that HGF treatment is another approach to increase the efficacy of direct reprogramming of fibroblasts towards induced EPCs by single ETV2 vector.
References
-
A.R.
Amini,
C.T.
Laurencin,
S.P.
Nukavarapu.
Differential analysis of peripheral blood- and bone marrow-derived endothelial progenitor cells for enhanced vascularization in bone tissue engineering. J Orthop Res.
2012;
30
:
1507-1515
.
-
T.
Asahara,
T.
Takahashi,
H.
Masuda,
C.
Kalka,
D.
Chen,
H.
Iwaguro,
Y.
Inai,
M.
Silver,
J.M.
Isner.
VEGF contributes to postnatal neovascularization by mobilizing bone marrow‐derived endothelial progenitor cells. The EMBO journal.
1999;
18
:
3964-3972
.
-
S.
Cherqui,
S.M.
Kurian,
O.
Schussler,
J.A.
Hewel,
J.R.
Yates,
Salomon
3rd.
Isolation and angiogenesis by endothelial progenitors in the fetal liver. Stem Cells.
2006;
24
:
44-54
.
-
K.D.
Choi,
J.
Yu,
K.
Smuga‐Otto,
G.
Salvagiotto,
W.
Rehrauer,
M.
Vodyanik,
J.
Thomson,
I.
Slukvin.
Hematopoietic and endothelial differentiation of human induced pluripotent stem cells. Stem Cells.
2009;
27
:
559-567
.
-
L.S.
Ferreira,
S.
Gerecht,
H.F.
Shieh,
N.
Watson,
M.A.
Rupnick,
S.M.
Dallabrida,
G.
Vunjak-Novakovic,
R.
Langer.
Vascular progenitor cells isolated from human embryonic stem cells give rise to endothelial and smooth muscle-like cells and form vascular networks in vivo. Circulation research.
2007;
101
:
286-294
.
-
J.K.
Han,
S.H.
Chang,
H.J.
Cho,
S.B.
Choi,
H.S.
Ahn,
J.
Lee,
H.
Jeong,
S.W.
Youn,
H.J.
Lee,
Y.W.
Kwon.
Direct conversion of adult skin fibroblasts to endothelial cells by defined factors. Circulation.
2014;
130
:
1168-1178
.
-
M.
Hirashima,
H.
Kataoka,
S.
Nishikawa,
N.
Matsuyoshi,
S.-I.
Nishikawa.
Maturation of embryonic stem cells into endothelial cells in an in vitro model of vasculogenesis. Blood.
1999;
93
:
1253-1263
.
-
M.
Hristov,
W.
Erl,
P.C.
Weber.
Endothelial progenitor cells: isolation and characterization. Trends Cardiovasc Med.
2003;
13
:
201-206
.
-
K.
Ishizawa,
H.
Kubo,
M.
Yamada,
S.
Kobayashi,
T.
Suzuki,
S.
Mizuno,
T.
Nakamura,
H.
Sasaki.
Hepatocyte growth factor induces angiogenesis in injured lungs through mobilizing endothelial progenitor cells. Biochem Biophys Res Commun.
2004;
324
:
276-280
.
-
C.
Kalka,
H.
Masuda,
T.
Takahashi,
W.M.
Kalka-Moll,
M.
Silver,
M.
Kearney,
T.
Li,
J.M.
Isner,
T.
Asahara.
Transplantation of ex vivo expanded endothelial progenitor cells for therapeutic neovascularization. roceedings of the National Academy of Sciences.
2000;
97
:
3422-3427
.
-
M.
Kondo,
A.J.
Wagers,
M.G.
Manz,
S.S.
Prohaska,
D.C.
Scherer,
G.F.
Beilhack,
J.A.
Shizuru,
I.L.
Weissman.
Biology of hematopoietic stem cells and progenitors: implications for clinical application. nnual review of immunology.
2003;
21
:
759-806
.
-
S.
Lee,
C.
Park,
J.W.
Han,
J.Y.
Kim,
K.
Cho,
E.J.
Kim,
S.
Kim,
S.-J.
Lee,
H.J.
An,
M.Y.
Sin.
Abstract 18205: Direct Reprogramming of Human Dermal Fibroblasts into Endothelial Cells Using a Single Transcription Factor. Circulation.
2014;
130
:
A18205-A18205
.
-
S.
Levenberg,
J.S.
Golub,
M.
Amit,
J.
Itskovitz-Eldor,
R.
Langer.
Endothelial cells derived from human embryonic stem cells. Proceedings of the national Academy of Sciences.
2002;
99
:
4391-4396
.
-
J.
Li,
N.F.
Huang,
J.
Zou,
T.J.
Laurent,
J.C.
Lee,
J.
Okogbaa,
J.P.
Cooke,
S.
Ding.
Conversion of human fibroblasts to functional endothelial cells by defined factors. Arterioscler Thromb Vasc Biol.
2013;
33
:
1366-1375
.
-
A.
Margariti,
B.
Winkler,
E.
Karamariti,
A.
Zampetaki,
T.N.
Tsai,
D.
Baban,
J.
Ragoussis,
Y.
Huang,
J.D.
Han,
L.
Zeng.
Direct reprogramming of fibroblasts into endothelial cells capable of angiogenesis and reendothelialization in tissueengineered vessels. Proc Natl Acad Sci U S A.
2012;
109
:
13793-13798
.
-
L.C.
Masek,
J.W.
Sweetenham.
Isolation and culture of endothelial cells from human bone marrow. r J Haematol.
1994;
88
:
855-865
.
-
L.E.
Mead,
D.
Prater,
M.C.
Yoder,
D.A.
Ingram.
Isolation and characterization of endothelial progenitor cells from human blood. Curr Protoc Stem Cell Biol Chapter.
2008;
2
:
Unit 2C 1
.
-
R.
Morita,
M.
Suzuki,
H.
Kasahara,
N.
Shimizu,
T.
Shichita,
T.
Sekiya,
A.
Kimura,
K.
Sasaki,
H.
Yasukawa,
A.
Yoshimura.
ETS transcription factor ETV2 directly converts human fibroblasts into functional endothelial cells. Proc Natl Acad Sci U S A.
2015;
112
:
160-165
.
-
C.
Park,
T.J.
Lee,
S.H.
Bhang,
F.
Liu,
R.
Nakamura,
S.S.
Oladipupo,
I.
Pitha-Rowe,
B.
Capoccia,
H.S.
Choi,
T.M.
Kim.
Injury-Mediated Vascular Regeneration Requires Endothelial ER71/ETV2. rterioscler Thromb Vasc Biol.
2016;
36
:
86-96
.
-
F.
Sanada,
Y.
Taniyama,
J.
Azuma,
K.
Iekushi,
N.
Dosaka,
T.
Yokoi,
N.
Koibuchi,
H.
Kusunoki,
Y.
Aizawa,
R.
Morishita.
Hepatocyte growth factor, but not vascular endothelial growth factor, attenuates angiotensin II-induced endothelial progenitor cell senescence. Hypertension.
2009;
53
:
77-82
.
-
K.
Schroder,
S.
Schutz,
I.
Schloffel,
S.
Batz,
I.
Takac,
N.
Weissmann,
U.R.
Michaelis,
M.
Koyanagi,
R.P.
Brandes.
Hepatocyte growth factor induces a proangiogenic phenotype and mobilizes endothelial progenitor cells by activating Nox2. Antioxid Redox Signal.
2011;
15
:
915-923
.
-
M.O.
Schupp,
M.
Waas,
C.Z.
Chun,
R.
Ramchandran.
Transcriptional inhibition of etv2 expression is essential for embryonic cardiac development. Dev Biol.
2014;
393
:
71-83
.
-
X.
Shi,
J.
Richard,
K.M.
Zirbes,
W.
Gong,
G.
Lin,
M.
Kyba,
J.A.
Thomson,
N.
Koyano-Nakagawa,
D.J.
Garry.
Cooperative interaction of Etv2 and Gata2 regulates the development of endothelial and hematopoietic lineages. Dev Biol.
2014;
389
:
208-218
.
-
M.B.
Song,
X.J.
Yu,
G.X.
Zhu,
J.F.
Chen,
G.
Zhao,
L.
Huang.
Transfection of HGF gene enhances endothelial progenitor cell (EPC) function and improves EPC transplant efficiency for balloon-induced arterial injury in hypercholesterolemic rats. Vascul Pharmacol.
2009;
51
:
205-213
.
-
K.
Takahashi,
S.
Yamanaka.
Induction of Pluripotent Stem Cells from Mouse Embryonic and Adult Fibroblast Cultures by Defined Factors. Cell.
2006;
126
:
663-676
.
-
T.
Takahashi,
C.
Kalka,
H.
Masuda,
D.
Chen,
M.
Silver,
M.
Kearney,
M.
Magner,
J.M.
Isner,
T.
Asahara.
Ischemia-and cytokine-induced mobilization of bone marrowderived endothelial progenitor cells for neovascularization. Nature medicine.
1999;
5
:
434-438
.
-
C.
Urbich,
S.
Dimmeler.
Endothelial progenitor cells characterization and role in vascular biology. irculation research.
2004;
95
:
343-353
.
-
P.
Van Pham,
N.B.
Vu,
H.T.
Nguyen,
O.T.
Huynh,
M.T.
Truong.
Significant improvement of direct reprogramming efficacy of fibroblasts into progenitor endothelial cells by ETV2 and hypoxia. Stem Cell Res.
2016;
Ther7
:
104
.
-
D.
Vittet,
M.-H.
Prandini,
R.
Berthier,
A.
Schweitzer,
H.
Martin- Sisteron,
G.
Uzan,
E.
Dejana.
Embryonic stem cells differentiate in vitro to endothelial cells through successive maturation steps. Blood.
1996;
88
:
3424-3431
.
-
Z.Z.
Wang,
P.
Au,
T.
Chen,
Y.
Shao,
L.M.
Daheron,
H.
Bai,
M.
Arzigian,
D.
Fukumura,
R.K.
Jain,
D.T.
Scadden.
Endothelial cells derived from human embryonic stem cells form durable blood vessels in vivo. Nature biotechnology.
2007;
25
:
317-318
.
-
X.
Yu,
M.
Song,
J.
Chen,
G.
Zhu,
G.
Zhao,
H.
Wang,
L.
Hunag.
Hepatocyte growth factor protects endothelial progenitor cell from damage of low-density lipoprotein cholesterol via the PI3K/Akt signaling pathway. Mol Biol Rep.
2010;
37
:
2423-2429
.
-
Y.J.
Zhou,
J.H.
Wang,
J.
Zhang.
Hepatocyte growth factor protects against apoptosis induced by advanced glycation end products in endothelial cells. Chin Med Sci J.
2006;
21
:
6-10
.
-
Y.J.
Zhou,
H.W.
Yang,
X.G.
Wang,
H.
Zhang.
Hepatocyte growth factor prevents advanced glycation end products-induced injury and oxidative stress through a PI3K/Aktdependent pathway in human endothelial cells. Life Sci.
2009;
85
:
670-677
.
-
G.
Zhu,
L.
Huang,
M.
Song,
Z.
Yu,
X.
Wu,
X.
Zhao,
J.
Jin,
G.
Zhao,
J.
Chen,
S.
Yu.
Over-expression of hepatocyte growth factor in smooth muscle cells regulates endothelial progenitor cells differentiation, migration and proliferation. Int J Cardiol.
2010;
138
:
70-80
.
Comments
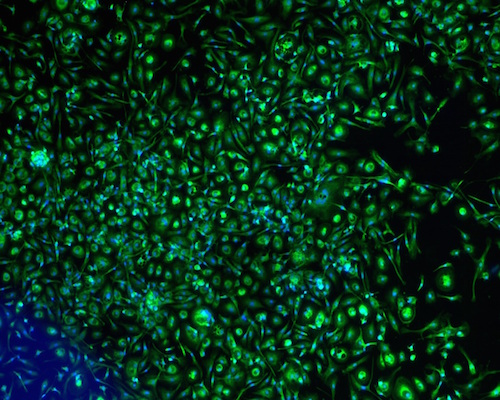
Downloads
Article Details
Volume & Issue : Vol 3 No 09 (2016)
Page No.: 836-843
Published on: 2016-10-03
Citations
Copyrights & License

This work is licensed under a Creative Commons Attribution 4.0 International License.
Search Panel
Pubmed
Google Scholar
Pubmed
Google Scholar
Pubmed
Google Scholar
Pubmed
Google Scholar
Pubmed
Google Scholar
Pubmed
Google Scholar
Pubmed
Search for this article in:
Google Scholar
Researchgate
- HTML viewed - 7659 times
- Download PDF downloaded - 1733 times
- View Article downloaded - 10 times