Abstract
Background: In biomedical research, silver nanoparticles have attracted the attention of many scientists in drug delivery and other applications. This study investigates the characterization of biologically synthesized nanoparticles and their efficacy on cancer cells.
Methods: The characterization of biologically produced silver nanoparticles was accomplished using UV:visible absorption spectroscopy, which showed the maximum absorption at 422 nm. The structural morphology was carried out by transmission electron microscope (TEM) and atomic force microscope (AFM), and the anticancer efficacy of the biosynthesized compound was investigated on two cancer cell lines, namely HT-29 (colon cancer) and EAC.
Results: The morphological and structural examination revealed spherical, three-dimensional nanoparticles ranging between 5 to 50 nm. The results of the anticancer experiment, which was conducted using an MTT assay, revealed that the prepared silver had excellent anti-cancer activity against both tested cells, that is EAC cancer and HT-29 (colon cancer) cell lines.
Conclusion: This study confirms that silver nanoparticles can be biologically synthesized using Rhizopus stolonifer and used as anticancer substances in cancer therapy and drug delivery.
Introduction
Silver nanoparticles have recently received considerable attention among scientists due to their unique biological properties1. The production and characterization of these nanoparticles is essential part to research2. Various methods have been used to produce silver nanoparticles, including physical, chemical, and newly established bio-mimetics processes3. However, these approaches are ecologically hazardous (especially chemical methods) and cause the quick agglomeration of nanoparticles, leading to the formation of large particles devoid of mono-dispersity4. Silver nanoparticles have been used for cancer therapy as anticancer materials and in drug delivery5. The succession of tumors has been attributed to a series of alterations in some cells leading to a certain type of unusual gene expression6, 7. Ubiquitously targeting cells inside malignant cells due to the inability of certain drugs to disperse proficiently may not be reasonable, as this possibly endorses multiple-drug resistance, resulting from resistance to the chemotherapeutic treatment of cancer cells8, 9. Furthermore, the expulsion of the drugs inevitably reduces healing activity such that tumor cells rapidly build up resistance to various drugs10.Silver nanoparticles (AgNPs) have been used considerably in cancer drug delivery. Owing to their unique properties and antimicrobial characteristics, many studies have used AgNPs’ inactive drug targeting and passive drug targeting11. Many recent studies have utilized AgNPs as a smart candidate for the targeted and controlled delivery of many different therapeutic agents, including proteins, lipids, carbohydrates, or even genes12, 13, 14, 15. Rajawat et al.16 used silver nanoparticles for the targeted delivery of breast cancer treatment and concluded that tumor volumes decreased significantly, with similar results in the positive control. Drug release from such nanoparticles can be elicited using internal or external stimuli17, 18.
Green chemistry using plant extracts and microorganisms has been steadily developed as an effective alternative to the environmentally harmful processes of silver nanoparticle synthesis19. Moreover, using microorganisms such as fungi in silver nanoparticle production is a low-cost process that helps to avoid generating. Recently, Roy et al.20 reviewed the green synthesized silver nanoparticles and their antimicrobial properties and revealed numerous types of microorganisms that could be utilized to produce different particle sizes with different functionalities in terms of antimicrobial and anticancer activity. The current research presents the utilization of R. stolonifer, one of the easiest fungi to grow, for silver nanoparticle production and studies the properties of bio synthesized nanoparticles in potential anticancer therapy.
Methods
Synthesis of silver nanoparticles
Rhizopus stolonifer was isolated from local soil samples and identified using microscopic and molecular techniques, and the fungi were inoculated into an appropriate amount of malt glucose yeast peptone broth (Oxoid, Basingstoke, Hampshire, UK), which was prepared as mentioned in Karbasian et al.21. The biomass was washed thoroughly with distilled water after 72 h of incubation at 25 °C. It was then placed in flasks containing 100 ml of distilled water and incubated again under the same conditions. Finally, the fungal biomass was filtered, and the filtrate was mixed in silver nitrate aqueous solution (Merck, Darmstadt, Germany) of final concentration (1 mM AgNO3) and then agitated at 40°C.
Characterization of silver nanoparticles
UV-visible absorption spectroscopy (Shimadzu UV2000) was used for the characterization of the prepared AgNPs; the experiment revealed the highest absorbance at 422 nm, indicating that the incubation of the AgNO3 solution (Merck, Darmstadt, Germany) with the occurrence of extracellular enzymes’ bio-reduction of AgNO3 solution had occurred. TEM (Japanese JEM-3000 F, Jeol) was also used for the structural characterization of the surface morphology of the nanoparticles using drop-coated film. Atomic Force Microscope (Japanese SMI3050SE FIB-AFM, Hitachi) was used to visualize and characterize the nanoparticles' three-dimensional structures.
Trypan blue dye exclusion assay
To determine the number of viable cells present in the cell suspension, the suspension was prepared with a fixed volume of cells. Furthermore, 50 μL of cell suspension was mixed with an equal volume of trypan blue (Merck, Darmstadt, Germany). The solution was mixed well using a micropipette, and then the living cells were counted using a hemocytometer (Hausser Scientific, Horsham, PA, USA), as live cells have a clear cytoplasm, whereas dead cells give a blue color22. The percentage of cell viability was then calculated using the following formula:
% viability = (live cell count / total cell count) x 100
MTT Assay
A spectrophotometer was used to measure the reduction of the yellow color of MTT tetrazolium dye (spectra max, Molecular devices) at 570 nm absorbance, and the IC50 values were determined by plotting % inhibition (from control) versus concentration. The living cells reduce the yellow-colored MTT reagent to a purple-colored formazan with the help of the mitochondrial reductase enzyme present in them. This experiment was conducted in the dark because the MTT reagent is sensitive to light. The absorbance of the colored solution was measured using a spectrophotometer at 560 nm.
Anti-cancer study
Two human cell lines were used: EAC and HT-29 (colon cancer); both were obtained from the National Centre for Cell Science (NCCS), Pune, India. MTT, Trypsin, EDTA, and DMEM (Dulbecco’s Modified Eagle’s Medium) were purchased from Sigma Chemicals Co, and 96-well flat-bottomed tissue culture plates were purchased from Tarson. Fetal bovine serum was purchased from Arrow labs. The cells were transferred to the 96-well plates (104 cells/well in 100 µl of medium) and incubated for about 24 h for attachment. After 72 h of incubation, the drug concentration was analyzed by constructing a graph for it against the percent cell toxicity and performing regression analysis.
Statistical analysis
Bonferroni’s post-hoc test single-way variance evaluation (ANOVA) of all groups was carried out every day.
Results
Characteristics of AgNPs
The results of UV-visible absorption spectroscopy showing maximum absorbance at 422 nm are presented in Figure 1. They indicate that the incubation of the silver nitrate solution with the occurrence of extracellular enzymes’ bio-reduction of the AgNO3 has occurred.
The TEM images indicate that the shape and size of the AgNPs are clearly spherical and nano (5 to 50 nm), respectively. Figure 2 presents the TEM of a single drop of AgNP-coated film. No agglomeration is noticed; all the particles are nicely separated, and the microscopic field shows size scales between 5 nm to 30 nm.
Figure 3 presents the images from the Atomic Force Microscope (AFM) for the prepared silver nanoparticles, showing the 3D structures and their arrangement on the film.
Concentration (µg/ml) | Viable cells count | Non-viable cells count | Inhibition (%) |
---|---|---|---|
Control | 196.1 | 28.76 | 13.02% |
8 | 104.6 | 211.26 | 68.13% |
4 | 117.6 | 183.16 | 62.12% |
2 | 179.26 | 168.26 | 49.19% |
1 | 223.76 | 76.1 | 26.03% |
0.5 | 235.1 | 34.6 | 13.21% |
Concentration (µg/ml) | Absorbance at 570 nm | Inhibition (%) |
---|---|---|
Control | 3.81 | ------ |
8 | 0.921 | 68.46 |
4 | 2.123 | 61.24 |
2 | 2.329 | 53.88 |
1 | 2.541 | 46.74 |
0.5 | 2.678 | 41.95 |
Cytotoxicity of AgNPs
The results in Table 1 present inhibition of the growth of esophageal squamous-cell carcinoma (EAC) cell lines with the use of the prepared AgNPs at different concentrations. The minimum concentration of AgNPs used was 0.5 μg/ml, which caused a reduction of 13.21% in the viability of the EAC cell line, whereas 1 μg/ml of biosynthesized AgNPs decreased the percentage of viable cells to 26.03%. This cytotoxic effect became even more significant with a 62.12% decrease in viable cells at 4 μg/ml of AgNPs. The maximum reduction of the EAC cell line viability was observed at 8 μg/ml concentration, which was 68.13%.
Figure 4 presents the graph of IC50 values, which show a cytotoxicity of more than 50% at the minimum concentration (2.15 μg/ml) of AgNPs. It can also be observed that this minimum concentration caused a reduction in the EAC cell lines by 50%.
Colon cancer cells (HT-29 cell lines) were mostly similar to EAC cell lines (Table 2); the maximum inhibition of cells was observed at a concentration of 8 μg/ml of AgNPs, which caused an inhibition of 68.46% of the cancer cells. In contrast, the minimum used dose of AgNPs (0.5 μg/ml) showed an inhibition of almost 42% of HT-29 cell lines; the reduction value was directly proportional to the increase in AgNP concentrations. The IC50 values of silver nanoparticles on colon cancer cell lines as presented in Figure 5 show that a concentration of 2 μg/ml, which is the middle concentration used in this study, could inhibit more than 50% of all the viable cancer cells.
Discussion
The use of fungi as reducing and stabilizing agents in the biogenic synthesis of silver nanoparticles is advantageous due to the production of large quantities of proteins, high yields, easy handling, and low toxicity of the residues23. Results of the present study indicate that green synthesized silver nanoparticles were spherical and 3Dranging in size between 5 and 50 nm. Similar results have been reported in other studies with varying particle sizes24, 25. Our findings for the structural characterization of nanoparticles are in accordance with Sadowski et al.26 and Maliszwaska et al.27, who utilized different microorganisms in the synthesis process. In a different study, Mukherjee et al.28 used the same methodology as used in this study and demonstrated an intense peak of 410 nm. Bio-reduction of silver nitrate (AgNO3) to synthesize silver in nano size and stabilization by capping agents are the two processes for manufacturing silver nanoparticles. The biological particles from fungi could conceivably play a role in the stabilization of silver nanoparticles29, 30. Cytotoxicity results of the present study were evaluated by inhibiting the growth of EAC cell lines using the prepared AgNPs at different concentrations. The maximum reduction of the EAC cell line was observed at 8 μg/ml concentration with an inhibition of over 68.13%, which was significantly higher than the gold nanoparticles used in the study conducted by Li et al.31 against the same cell line. The cytotoxicity of biologically prepared AgNPs was determined using MTT; the results indicate that the AgNPs were competent to reduce the viability of the Dalton's Lymphoma Ascites cells in a dose-dependent manner; the higher the AgNPs’ concentration, the more is the reduction rate. These results confirm findings by Priya et al.32, who synthesized AgNPs using a chitosan-mediated approach and achieved dose-dependent inhibition. They concluded that silver nanoparticles caused the up-regulation of certain genes, leading to cell death via apoptosis. In this study, the effectiveness of the biosynthesized AgNPs on tumor cells was analyzed after six hours of treatment at concentrations of 500 nM and higher. Biologically prepared nanoparticles at 500 nM dramatically inhibited the viability and growth of Dalton's Lymphoma Ascites cells by more than 50%, which was chosen as the IC50. The toxicity to the cells increased when exposed for a longer period of time, confirming gene alteration even in the still viable cells.
Conclusions
The utilization of microorganisms for metal nanoparticle synthesis is a comparatively modern innovation for a cleaner and cost-effective synthesis method. The easy scalability of such a method may help to reduce the costs of production of viable anticancer agents such as silver nanoparticles. Accordingly, this research has shown the green process for the production of AgNPs using R. stolonifer. Our study has shown maximum absorbance at 422 nm with UV-Visible absorption spectroscopy. The size and shape of the silver nanoparticles were revealed by Transmission Electron Microscope as 5 to 50 nm and spherical, respectively. The silver nanoparticles produced by the biological method have extensive applications, including cancer therapy. This study shows the activity of biologically synthesized silver nanoparticles on the inhibition of cancer cells.
Abbreviations
AFM: Atomic Force Microscope
AgNPs: Silver Nanoparticles
DMEM: Dulbecco’s Modified Eagle’s Medium
EAC: Esophageal squamous-cell carcinoma
NCCS: National Centre for Cell Science
R. stolonifer: Rhizopus stolonifer
TEM: Transmission Electron Microscope
Acknowledgments
The authors would like to thank the collaboration between Lincoln University College, Selangor, Malaysia and the Universiti Sains Malaysia, Penang, Malaysia that made this work possible.
Author’s contributions
All authors contributed equally to this work.
Funding
None.
Availability of data and materials
Data and materials used and/or analyzed during the current study are available from the corresponding author on reasonable request.
Ethics approval and consent to participate
Not applicable.
Consent for publication
Not applicable.
Competing interests
The authors declare that they have no competing interests.
References
-
Singh
R.,
Shedbalkar
U.U.,
Wadhwani
S.A.,
Chopade
B.A.,
Bacteriagenic silver nanoparticles: synthesis, mechanism, and applications. Applied Microbiology and Biotechnology.
2015;
99
(11)
:
4579-93
.
View Article PubMed Google Scholar -
Banu
A.,
Rathod
V.,
Ranganath
E.,
Silver nanoparticle production by Rhizopus stolonifer and its antibacterial activity against extended spectrum β-lactamase producing (ESBL) strains of Enterobacteriaceae. Materials Research Bulletin.
2011;
46
(9)
:
1417-23
.
View Article Google Scholar -
Zhang
X.F.,
Liu
Z.G.,
Shen
W.,
Gurunathan
S.,
Silver nanoparticles: synthesis, characterization, properties, applications, and therapeutic approaches. International Journal of Molecular Sciences.
2016;
17
(9)
:
1534
.
View Article PubMed Google Scholar -
Wei
L.,
Lu
J.,
Xu
H.,
Patel
A.,
Chen
Z.S.,
Chen
G.,
Silver nanoparticles: synthesis, properties, and therapeutic applications. Drug Discovery Today.
2015;
20
(5)
:
595-601
.
View Article PubMed Google Scholar -
Bukhari
N.,
Joseph
J.P.,
Hussain
S.S.,
Khan
M.A.,
Wakim
M.J.,
Yahya
E.B.,
Prevalence of Human Papilloma Virus Sub Genotypes following Head and Neck Squamous Cell Carcinomas in Asian Continent, A Systematic Review Article. Asian Pacific Journal of Cancer Prevention.
2019;
20
(11)
:
3269-77
.
View Article PubMed Google Scholar -
Almashgab
A.M.,
Yahya
E.B.,
Banu
A.,
The Cytotoxicity Effects of Outer Membrane Vesicles Isolated From Hospital and Laboratory Strains of Pseudomonas Aeruginosa on Human Keratinocyte Cell Line. Malaysian Journal of Science.
2020;
39
(3)
:
45-53
.
View Article Google Scholar -
Siegel
R.L.,
Miller
K.D.,
Jemal
A.,
Cancer statistics, 2019. CA: a Cancer Journal for Clinicians.
2019;
69
(1)
:
7-34
.
View Article PubMed Google Scholar -
Khalil
H.P.,
Jummaat
F.,
Yahya
E.B.,
Olaiya
N.G.,
Adnan
A.S.,
Abdat
M.,
A review on micro-to nanocellulose biopolymer scaffold forming for tissue engineering applications. Polymers.
2020;
12
(9)
:
2043
.
View Article PubMed Google Scholar -
Yahya
E.B.,
Evaluation of in-vitroantibacterial activity of aqueous and alcoholic extracts of the peels punica granatum and olea europaea leaves. J. Sci. Technol. Medical Science.
2018;
2
(1)
:
36-44
.
-
Abdul Khalil
H.P.,
Adnan
A.S.,
Yahya
E.B.,
Olaiya
N.G.,
Safrida
S.,
Hossain
M.S.,
A Review on plant cellulose nanofibre-based aerogels for biomedical applications. Polymers.
2020;
12
(8)
:
1759
.
View Article PubMed Google Scholar -
Yahya
E.B.,
Jummaat
F.,
Amirul
A.A.,
Adnan
A.S.,
Olaiya
N.G.,
Abdullah
C.K.,
A review on revolutionary natural biopolymer-based aerogels for antibacterial delivery. Antibiotics (Basel, Switzerland).
2020;
9
(10)
:
648
.
View Article PubMed Google Scholar -
Karuppaiah
A.,
Rajan
R.,
Hariharan
S.,
Balasubramaniam
D.K.,
Gregory
M.,
Sankar
V.,
Synthesis and Characterization of Folic Acid Conjugated Gemcitabine Tethered Silver Nanoparticles (FA-GEM-AgNPs) for Targeted Delivery. Current Pharmaceutical Design.
2020;
26
(26)
:
3141-6
.
View Article PubMed Google Scholar -
Gholamali
I.,
Asnaashariisfahani
M.,
Alipour
E.,
Silver nanoparticles incorporated in pH-sensitive nanocomposite hydrogels based on carboxymethyl chitosan-poly (vinyl alcohol) for use in a drug delivery system. Regenerative Engineering and Translational Medicine.
2020;
6
(2)
:
138-53
.
View Article Google Scholar -
Ansar
S.,
Tabassum
H.,
Aladwan
N.S.,
Naiman Ali
M.,
Almaarik
B.,
AlMahrouqi
S.,
Eco friendly silver nanoparticles synthesis by Brassica oleracea and its antibacterial, anticancer and antioxidant properties. Scientific Reports.
2020;
10
(1)
:
18564
.
View Article PubMed Google Scholar -
Yahya
E.B.,
Alqadhi
A.M.,
Recent trends in cancer therapy: A review on the current state of gene delivery. Life Sciences.
2021;
269
:
119087
.
View Article PubMed Google Scholar -
Rajawat
S.,
Malik
M.M.,
Targeted Delivery of Colloidal Silver for MCF-7 Breast Cancer Treatment. Current Drug Delivery.
2020;
17
(7)
:
613-21
.
View Article PubMed Google Scholar -
Riley
R.S.,
June
C.H.,
Langer
R.,
Mitchell
M.J.,
Delivery technologies for cancer immunotherapy. Nature Reviews. Drug Discovery.
2019;
18
(3)
:
175-96
.
View Article PubMed Google Scholar -
Yahya
E.,
Abdulsamad
M.A.,
In-vitro Antibacterial Activity of Carbopol-Essential Oils hydrogels. Journal of Applied Science {&} Process Engineering.
2020;
7
(2)
:
564-71
.
View Article Google Scholar -
Castillo-Henríquez
L.,
Alfaro-Aguilar
K.,
Ugalde-Álvarez
J.,
Vega-Fernández
L.,
Montes de Oca-Vásquez
G.,
Vega-Baudrit
J.R.,
Green Synthesis of Gold and Silver Nanoparticles from Plant Extracts and Their Possible Applications as Antimicrobial Agents in the Agricultural Area. Nanomaterials (Basel, Switzerland).
2020;
10
(9)
:
1763
.
View Article PubMed Google Scholar -
Roy
A.,
Bulut
O.,
Some
S.,
Mandal
A.K.,
Yilmaz
M.D.,
Green synthesis of silver nanoparticles: biomolecule-nanoparticle organizations targeting antimicrobial activity. RSC Advances.
2019;
9
(5)
:
2673-702
.
View Article Google Scholar -
Karbasian
M.,
Atyab
S. M.,
Siadat
S. D.,
Momen
S. B.,
Norouzian
D.,
Optimizing Nano-silver Formation by Fusarium oxysporum PTCC 5115 Employing Response Surface Methodology. American Journal of Agricultural and Biological Sciences.
2008;
3
(1)
:
433-437
.
View Article Google Scholar -
Hussein
R.A.,
Mohsin
A.J.,
Trypan Blue Exclusion Assay Verifies in Vitro Cytotoxicity of New Cis-Platinum (II) Complex in Human Cells. Baghdad Science Journal.
2019;
16
(3)
:
555-559
.
-
Guilger-Casagrande
M.,
de Lima
R.,
Synthesis of silver nanoparticles mediated by fungi: a review. Frontiers in Bioengineering and Biotechnology.
2019;
7
:
287
.
View Article PubMed Google Scholar -
Feroze
N.,
Arshad
B.,
Younas
M.,
Afridi
M.I.,
Saqib
S.,
Ayaz
A.,
Fungal mediated synthesis of silver nanoparticles and evaluation of antibacterial activity. Microscopy Research and Technique.
2020;
83
(1)
:
72-80
.
View Article PubMed Google Scholar -
Hulikere
M.M.,
Joshi
C.G.,
Characterization, antioxidant and antimicrobial activity of silver nanoparticles synthesized using marine endophytic fungus-Cladosporium cladosporioides. Process Biochemistry (Barking, London, England).
2019;
82
:
199-204
.
View Article Google Scholar -
Sadowski
Z.,
Synthesis of silver nanoparticles using microorganisms. Materials Science Poland.
2008;
26
(2)
:
419-24
.
-
Maliszewska
I.,
Sadowski
Z.,
Synthesis and antibacterial activity of of silver nanoparticles. Journal of Physics: Conference Series.
2009;
146
:
012024
.
View Article Google Scholar -
Mukherjee
P.,
Roy
M.,
Mandal
B.P.,
Dey
G.K.,
Mukherjee
P.K.,
Ghatak
J.,
Green synthesis of highly stabilized nanocrystalline silver particles by a non-pathogenic and agriculturally important fungus T. asperellum. Nanotechnology.
2008;
19
(7)
:
075103
.
View Article PubMed Google Scholar -
Yahya
E.,
Almashgab
A.M.,
Abdulsamad
M.A.,
Allaq
A.A.,
Alqadhi
A.M.,
Garatem
F.M.,
Evaluation the Effect of Microwave Radiation on Gram Positive and Negative Bacteria. Journal of Chemistry and Nutritional Biochemistry.
2021;
2
(1)
:
39-45
.
View Article Google Scholar -
Tripathi
A.,
Liu
S.,
Singh
P.K.,
Kumar
N.,
Pandey
A.C.,
Tripathi
D.K.,
Differential phytotoxic responses of silver nitrate (AgNO3) and silver nanoparticle (AgNps) in Cucumis sativus L. Plant Gene.
2017;
11
:
255-64
.
View Article Google Scholar -
Li
Y.,
Gobin
A.M.,
Dryden
G.W.,
Kang
X.,
Xiao
D.,
Li
S.P.,
Infrared light-absorbing gold/gold sulfide nanoparticles induce cell death in esophageal adenocarcinoma. International Journal of Nanomedicine.
2013;
8
:
2153-61
.
PubMed Google Scholar -
Priya
K.,
Vijayakumar
M.,
Janani
B.,
Chitosan-mediated synthesis of biogenic silver nanoparticles (AgNPs), nanoparticle characterisation and in vitro assessment of anticancer activity in human hepatocellular carcinoma HepG2 cells. International Journal of Biological Macromolecules.
2020;
149
:
844-52
.
View Article PubMed Google Scholar
Comments
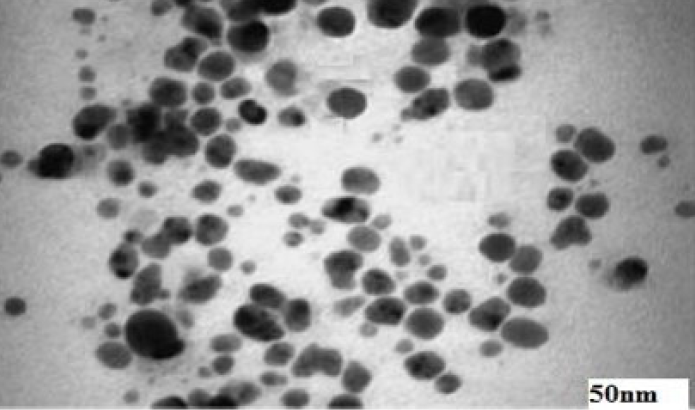
Article Details
Volume & Issue : Vol 8 No 8 (2021)
Page No.: 4476-4482
Published on: 2021-08-26
Citations
Copyrights & License

This work is licensed under a Creative Commons Attribution 4.0 International License.
Search Panel
Pubmed
Google Scholar
Pubmed
Google Scholar
Pubmed
Search for this article in:
Google Scholar
Researchgate
- HTML viewed - 6869 times
- PDF downloaded - 1446 times
- XML downloaded - 0 times