Abstract
Introduction: Mesenchymal stem cells (MSCs) have been known to have angiogenic potency, particularly for wound healing. However, it is such a cumbersome procedure to obtain a large amount of MSCs due to the culture process. The stromal vascular fraction (SVF), or so called nonexpanded MSCs, of adipose tissue is gaining increased interest in the field of cell therapy. An optimized method that takes only 2 hours to produce a large amount of stromal cells from adipose tissue has been developed. Burn wound model was used to assess the efficacy of SVF produced by our method. This study aimed to evaluate the efficacy of SVF compared to MSCs.
Methods: Male Sprague-Dawley rats with second degree burn wound were divided into the study groups: SVF, MSCs and saline control. The wound was photographed and evaluated weekly up to 5 weeks. The comparative analysis consisted of healing time, histology of skin tissue, as well as rat and human vascular endothelial growth factor (VEGF) expression.
Results: In this study, wounds treated with SVF and MSCs were smaller after 14-21 days, compared to the untreated group. Expression of rat VEGF in SVF and MSC- treated groups after seven days post-wounded were also higher than the untreated group. No human VEGF was found expressed in the skin biopsies.
Conclusion: This suggests that SVF and MSCs promote wound healing via a paracrine effect. The results suggest that SVF may be useful for wound healing and may be used as a promising alternative to MSC-based therapy.
INTRODUCTION
Adipose tissue is a source of multipotent mesenchymal stem cells (MSCs), much like bone marrow. The tissue is derived from mesenchyme and contains several types of cells, such as pericytes, stem cells, endothelial cells, and their progenitors, smooth muscle cells, adipocytes and pre-adipocytes, and hematopoietic cells1. Zuk et al. termed the cells derived from adipose tissue as a stromal vascular fraction (SVF)2.
Determining optimal cell dose is important for successful cell therapy. Koga and his coworkers demonstrated that a dosage of 50 million MSCs per mL injected into cartilage defects in rabbit was a superior dose than 1 million MSCs per mL for cartilage repair3. This result suggests that the amount of MSCs needed for humans might be somewhat larger than that reported for rabbits, especially for intravenous administration. The MSCs, whether they are bone marrow-derived or adipose tissue-derived, are often difficult to obtain in large amounts upon harvest. On the other hand, SVF can be easily obtained in a larger amount from lipoaspirates within only two hours, as has been previously demonstrated by our group4. Therefore, it seems promising to use SVF as an alternative to MSCs for clinical application.
In this study, we investigated the efficacy of SVF for burn wound healing in a rat model, with adipose-derived MSCs (AD-MSCs) used in the comparison. Rat and human VEGF expression were measured to evaluate the angiogenic potency of SVF and MSCs.
METHODS
This study was conducted in a facility at the Animal Hospital of Bogor Agricultural Institute in compliance with an animal protocol that had been reviewed and approved by the Health Research Ethics Committee (at the University of Indonesia) and Cipto Mangunkusumo Hospital (HREC-FMUI/CMH), with letter no. 625/UN2.F1/ETIK/2016. Twenty-eight male Sprague-Dawley rats, aged 8−10 weeks old and with the body weight of 100-175 grams, were randomized into three groups: human SVF-treated group, MSC-treated group, and saline-treated group (as control). For the SVF collection, donors were patients of Klinik Hayandra Hospital with various health conditions who had signed an informed consent and had been recruited consecutively based on their treatment registration date. SVF was collected from each patient. Some of SVFs from each donor were cultured to obtain passage 1 MSCs to be used in the study.
SVF Preparation
SVF was prepared from fresh human lipoaspirates using the previously described method4. Tissue-dissociation enzyme of H-Remedy was added to lipoaspirates in 50 mL tubes and incubated for 1 hour at 37ºC. After incubation, low glucose Dulbecco's Modified Eagle's Medium (DMEM) containing 4 mM L-glutamine (Gibco/ Thermo Fisher Scientific, Waltham, MA) was added to inactivate the enzyme. Digested lipoaspirates in tubes were centrifuged for 5 minutes at 600 g. Supernatants in all tubes were discarded, and SVF as cell pellets were collected, mixed with red cell buffer lysis, and incubated for 10 minutes at room temperature. After centrifugation, SVF was washed twice with phosphate buffer saline (PBS) of pH 7.4. SVF was diluted in saline solution and kept at 4˚C for 1−3 days prior to use.
Culture of MSCs
Some of the SVFs from donors were cultured in 25 cm2 flask at a cell density of 125,000 cells/flask until cells were attached at 37˚C. A combination of low glucose DMEM containing 4 mM L-glutamine (Gibco/ Thermo Fisher Scientific, Waltham, MA) supplemented with 10% FBS (Gibco), 1% antibiotic/antimycotic solution (10,000 units/mL of penicillin, 10,000 µg/mL of streptomycin, and 25 µg/mL of Amphotericin B) (Gibco), and 0.05 ng/mL L-ascorbic acid was added in the culture medium. The medium was replenished with fresh medium every 2−3 days. Cells were sub-cultured after they reached 80% confluency. Passage 1 MSCs were harvested and stored in saline solution at 4˚C only for 1−3 days before use.
Characterization of MSCs
Cell Differentiation Assay
MSCs (2 x 104 cells) were seeded in 24-well plates and cultured. After cells reached 80% confluency, the culture medium was replaced with osteogenic, chondrogenic, and adipogenic medium, as described by the manufacturers (STEMPRO Osteogenesis, Chondrogenesis, and Adipogenesis Differentiation Kit; Gibco/ Thermo Fisher Scientific, Waltham, MA). Adipocyte, chondrocyte, and osteocytes can be detected by Oil Red O (Sigma-Aldrich, Louis St, MO), Alcian Blue (Sigma-Aldrich), and Alizarin Red (Sigma-Aldrich) staining, respectively.
Phenotyping Analysis
Phenotyping analysis from two randomly selected MSC samples was done using 3-laser and 10-channel multiparameter flow cytometer (Miltenyi Biotec Inc., Auburn, CA). Cell surface markers used were APC-labeled mouse anti-human CD73, FITC-labeled mouse anti-human CD90, PerCP Cy5.5-labeled mouse anti-human CD105 (as a positive MSC marker), and a cocktail marker consisting of CD34, CD45, CD11b, CD19, and HLA-DR (PE-conjugated) as positive hematopoietic cells markers (Becton Dickinson, Franklin Lakes, NJ). Antibody titration was performed before the analysis to determine the antibody amount and concentration that produces the lowest signal of the negative population and highest signal of the positive population- in order to reduce nonspecific antibody binding. Unstained cells and isotype antibody were used as control. Debris was excluded from analyzed cells. The results represented a collection of 10.000 events per analyzed sample.
Second Degree Wound Healing Model and Transplantation
A wound healing model by Zhang et al.5 was used as a reference with minor modifications. After 1 week of the adaptation period, rats were injected intraperitoneally with ketamine and xylazine (0.1 mL/20 g of rat body weight). The shaved back of the rats were contacted for 4 seconds with a flat-round bottom stainless steel (200 g) that have been previously incubated in boiled water for 5 minutes. Five hundred µL of SVF in saline solution (4 x 107 cells per rat) or MSCs in saline solution (4 x 105 cells per rat) from the same donors, or saline as a control, were injected intradermally to the back of the rat.
Transplantations were done in two rounds: the first round was for SVF transplantation, and the second round was for MSC transplantation. There were 6−8 weeks apart between SVF and MSC transplantation for MSC culture. However, in this study, we were not able to obtain 4 x 105 MSCs from 4 of 8 donors due to long population doubling time, but from each of the rest of the donors, we were able to culture MSCs in sufficient numbers for transplantation in two rats. In this study, one rat received SVF/MSCs from one donor, but there were SVF/MSCs from 4 donors for which each was transplanted in two rats. The total rats receiving SVF, MSCs, and saline injection were 12, 8, and 8, respectively. Rats were fed ad libitum up to 5 weeks. For real-time polymerase chain reaction (PCR) analysis, 4 rats in the SVF-treated and 4 rats in the MSCs-treated groups, as well as 2 rats in the control group, were killed on day 7 and the skin collected. After 5 weeks (35 days), there were 8, 4, and 6 rats in the SVF, MSCs, and control group, respectively, that remained for the weekly micro- and macro-scopic evaluations.
Wound Healing Analysis
Macroscopic evaluation
The wound was inspected weekly, photographed and evaluated by image analysis software (Image J; National Institute of Health, Bethesda, MD, USA) to measure the wound area by two blinded investigators. The percentage of wound area was quantified by dividing the measured wound area post-wounded with the baseline wound area (taken on day 0) to measure closure rates between treatment groups.
Microscopic evaluation
At the end of the study (week 5), rats were euthanized. All rats were killed using the cervical dislocation technique. The entire wound with intact skin was collected and subjected to histological analysis by hematoxylin-eosin (H&E) staining. Tissue regeneration was analyzed based on re-epithelialization, the presence of skin appendages (hair follicle and sebaceous gland), structure, and thickness of the dermis.
Real-time Analysis of VEGF Expression
At day 7, wounded skin tissue of 2−4 rats from each group was randomly collected and subjected to measurement of rat and human VEGF expression by real-time PCR method.
In brief, total ribonucleic acid (RNA) from tissue was extracted using QIAamp RNA Blood Mini Kit (Qiagen, Germantown, MD, USA) according to the manufacturer’s protocol. The concentration and quality of RNA were measured using a spectrophotometer. Complementary DNA (cDNA) was synthesized from RNA by QuantiTect Reverse Transcription Kit (Qiagen). The resultant cDNA was subjected to quantitative real-time PCR (qPCR) performed using QuantiTect SYBR Green PCR Kit (Qiagen). Primer sequences were designed to be specific for rats or humans. The primer sequences of VEGF from rat were 5’-GTGTGGTCTTTCGTCCTTCTTA-3’ (forward) and 5’-GTTTGTCGTGTTTCTGGAAGTG-3’ (reverse), and for human were 5’-GAGCTTCCTACAGCACAACA-3’ (forward) and 5’-CCAGGACTTATACCGGGATTTC-3’ (reverse). β-actin primer sequences were used as internal controls and were 5’-GTGTGGATTGGTGGCTCTATC-3’ (forward) and 5’-CAGTCCGCCTAGAAGCATTT-3’ (reverse) for the rat, and 5’-CACTCTTCCAGCCTTCCTTC-‘3 (forward) and 5’-GTACAGGTCTTTGCGGATGT-3’ (reverse) for human. The following qPCR protocol was employed: 40 cycles of denaturation at 94ºC for 15 sec, annealing at 57 ºC for 30 sec, and extension at 72ºC for 30 sec. The single melting curve results confirmed there was no unspecific reaction during amplification. Relative mRNA expression was quantified by dividing gene expression from SVF or MSC-treated samples to untreated control sample.
Statistics
Data were tested for normal distribution and equal variance. Differences between experimental groups were then calculated by analysis of variance (ANOVA) followed by the least significant difference post hoc analysis or Kruskal-Wallis, followed by Mann-Whitney U test for normal distribution with equal variance and non-normal distribution or unequal variance data. ANOVA for repeated measures was applied to test for time effects within each experimental group. Wound areas were represented as mean ± SEM. Statistical significance was accepted if p< 0.05.
RESULTS
Donor Demographics
SVF was obtained from 6 female and 2 male donors that were SVF patients with various health conditions (5 patients with no pathological condition, 2 patients with musculoskeletal disorders, and 1 patient with autism). The mean age of the donor was 59±14 years old, with a range of 34−75 years old.
Cultured MSCs from Isolated SVFs
According to the International Society of Cellular Therapy (ISCT), most MSCs should express high levels of CD73, CD90 and CD105 (more than 95%) and low (less than 2%) levels of CD34, CD45, CD11b, CD19, and HLA-DR, as these are expressed in hematopoietic cells. In this study, 99% of our plastic adherent, fibroblast-like MSCs expressed CD73 and CD90, and did not express CD34, CD45, CD11b, CD19 or HLA-DR (Figure 1). However, the MSCs did not express CD105 as high as MSCs derived from bone marrow, as defined by the ISCT.
All of our cultured MSCs were shown to be able to differentiate into adipocytes, osteocytes and chondrocytes over the 4-day induction period by common differentiation medium, as shown in Figure 2.
Efficacy of SVF and MSCs in Wound Healing
The efficacy of each treatment was analyzed based on the percentage of wound area measured over a 5-week period, as presented in Figure 4. On day 7, the MSC-treated group had an average wound area that was larger than baseline. After 7 days and onward, the wound area was reduced in all groups, and there was no statistical difference observed between the treated groups (SVF or MSCs) versus the untreated group. The wound closure rate was faster in SVF-treated or MSC-treated group, compared to untreated group, during the 14−21 day period; the wound in the MSC-treated group closed slightly faster than that of the SVF-treated group. However, there was no significant difference between MSC- or SVF-treated groups on day 21. On day 28, the area of the wound was reduced to about 20% in all groups. At the end of the study, the wound area in the MSC-treated group was significantly larger than that of the SVF-treated or untreated group. The macroscopic change of the wound, weekly up to 35 days post-wound, is shown in Figure 3.
Histopathological Examination of Skin Tissue
Re-epithelialization
After 35 days, the epidermis layer in the SVF- and MSC-treated groups were thicker than that of the untreated group. The average length of skin layer that had not been covered by epithelial layer in the SVF-treated group was 6.73±0.26 mm, while in the MSC-treated group it was 6.68±0.98 mm. The untreated group had the longest layer that was uncovered by epithelium (10.41±5.01 mm).
Dermis layer
After 35 days, there was no significant difference in thickness of dermal layer between the groups (993.15±129.09 µm for saline, 1148.78±34.30 µm for SVF treated group, and 1108.79±166.53 µm for MSCs treated group; p>0.05). However, the dermis layer in the SVF- and MSC-treated groups were dense compared to that of the untreated group (Figure 5).
Skin Appendages
Hair follicles and sebaceous glands were present in all groups. However, hair follicles and sebaceous glands were more prominent (and each of these was larger) in SVF- and MSC- treated groups compared to the untreated group, especially in skin sections where the skin was completely covered by epithelium.
Measurement of VEGF Expression in Skin Tissue
We measured the expression of VEGF, an angiogenic growth factor that plays a key role in wound healing. Samples were obtained after 7 days post-wounded when the proliferative phase was assumed. On day 7, VEGF expressions in treated groups (SVF and MSCs) were increased compared to the untreated group but were not significantly different when statistically examined. There was no significant difference found between VEGF expression in SVF- and MSC-treated groups (1.74±1.50 for SVF-treated group versus 1.79±0.40 for MSC-treated group, p=0.96) (Figure 6).
We also analyzed the expression of human VEGF in skin tissue samples to detect the presence of VEGF from SVF and MSCs. However, in this study, we did not find any expression of VEGF from SVF or MSC donor in skin tissue taken on day 7 post-wounded.
DISCUSSION
We demonstrated that SVF could be easily isolated from adipose tissue. By culturing the cells, we observed that MSCs were present in SVF in this study. Our cultured MSCs from donors aged 50−60 years old showed fibroblast-like morphology and ability to adhere to plastic, similar to other reported studies6,7. Expression of CD73 and CD90 in our cultured MSCs was observed in almost all cells (close to 100%). Our results are similar to those reported by the ISCT to be expressed in bone marrow-derived MSCs8. The ISCT describes that more than 95% of MSCs express CD105 (very high percentage). However, in this study, we found that our highest percentage of CD105+ cells was only 70%. Another study also found similar results; the donor ages were from 50−60 years old, and the percentage of MSCs with CD105 expression was about 70−80%9. No clear mechanism has been reported for the lower CD105 expression in MSCs. However, since the ISCT set is the criteria for bone-marrow derived MSCs, CD105 expression in bone-marrow derived MSCs may differ from those expressed in adipose-derived MSCs. Indeed, the differences in surface marker expression of MSCs derived from bone marrow versus adipose tissue have been reported previously by other studies9,10.
In this study, we found that SVF and MSCs from elderly donors promote second degree burn wound healing during the 14−21 day period post-wound in the rat model, compared to untreated rats. Both SVF and MSC treatments were able to increase the expression of VEGF, an angiogenic growth factor that is important for wound healing. This paracrine effect has been reported in other wound healing studies. Hosni and coworkers reported that TGF-β1, another important angiogenic growth factor, was increased in rat skin after MSC treatment11. Similar results have also been reported by Lee et al.12. Moreover, Carolina et al. reported that plasma TGF-β level was increased in severe burn rat treated with MSCs13. These data concluded that upregulation of angiogenic growth factors, such as VEGF and TGF-β, are needed in the inflammatory and proliferative phases during wound healing, as well as in neo-vascularization and skin remodeling14. However, we were unable to find a significant difference between SVF- and MSC-treated groups. We also observed that the standard deviation of VEGF levels in SVF-treated group was higher compared to that of the MSC-treated group. These are probably due to the heterogeneity of cells within SVFs, compared to MSCs, which have already gone through the purification process (eliminating non-adherent cells and enriching for cells that are positive for CD73, CD90, and CD105 expression) during cell passaging. We also did not observe any expression of human VEGF on skin tissue after 7 days post-wound. It has known that MSCs are often stressed and begin to die after being transplanted, releasing various cytokines and growth factors beneficial for wound healing15.
In this study, we did not find any significant difference between the SVF- and MSC-treated groups. This may be due to the heterogeneity of SVF composition and MSC-associated biological functions, such as CD105 expression and MSC proliferation. In regards to SVF composition, endothelial cells, progenitor cells, and immune cells within SVF may have synergistic interactions with MSCs for promoting wound healing. Another possible reason is that MSCs in this study were obtained from other elder donors with unstandardized health conditions.
Our flow cytometry data analysis revealed that MSCs in this study have CD105 expression that is less than 95%. CD105 is an accessory protein that interacts with the receptor for pro-angiogenic TGF-β. Therefore, lower CD105 expression by MSCs may reduce angiogenic potency resulting in lower wound healing rate, as reported in this study. Another study reported that MSCs with CD105 expression more significant than 95% and combined with platelet-rich plasma (PRP) could rapidly heal damaged mouse knee joints when compared to control or PRP alone16. These data suggest that for clinical applications, MSCs should express CD105 at a higher level than MSCs in this study. High CD105 expression in MSCs can be achieved from healthy and young donors. Therefore, selecting MSC donors is essential in successful cell therapy; this was not applied in our study and might represent and explain the limitation of our study. However, a study that is designed to mimic this actual condition of selecting healthy and young donors cannot be done in autologous stem cell therapy.
In some conditions, such as the case of autologous therapy for the elderly or people with diabetes or other pathological conditions, effective cell therapy still may be achieved by increasing the injected MSC number. However, this approach presents a new problem. For instance, MSCs from elderly or non-healthy patients have a low proliferative capacity, as has been observed in past studies9,17,18. Therefore, it is necessary to culture MSCs for more extended periods. However, extended in vitro culture of MSCs have the potential to reduce the stemness, multipotency and therapeutic effectiveness in cartilage repair of MSCs when combined with a 3D matrix19. Therefore, advanced culture methods or technologies are needed to maintain the quality of MSCs and to prevent MSCs from undergoing senescence or apoptosis. On the other hand, SVF can be easily obtained in large numbers, varying between 1−10 billion cells from 50−250 cc of adipose tissue, from patients of any age and health condition (except for malignancies) within 2 hours. These have been demonstrated by our previous study4.
CONCLUSION
Both SVF and MSCs can promote wound healing in second degree burn wound model. SVF can be used as a promising alternative to MSCs as it is easier and faster to isolate a larger number of SVF compared to MSC preparation.
Abbreviations
AD-MSCs: adipose-derived MSCs
ANOVA: analysis of variance
cDNA: complementary DNA
DMEM: Dulbecco’s Modified Eagle’s Medium
ISCT: international society of cellular therapy
MSCs: mesenchymal stem cells
PBS: phosphate buffer saline
PRP: platelet-rich plasma
qPCR: quantitative polymerase chain reaction
RNA: ribonucleic acid
SVF: stromal vascular fraction
VEGF: vascular endothelial growth factor
Competing Interests
The authors indicated no potential conflicts of interest.
Authors' Contributions
Conception and design: KRN, IRA, IRI; contribution in cell culture works: SSH, IAI, TWI; data collection and entry: SSH, IRA, IRI, IAI, TWI; data analysis and interpretation: KRN, IRA, IRI; manuscript writing: KRN, IRA; final approval of manuscript: KRN, IRI, IRA.
Acknowledgments
The authors would like to thank Prof. dr. Jeanne Adiwinata Pawitan, MS, PhD for guiding the laboratory work and Komite Akreditasi Nasional-certified Laboratorium Terpadu Fakultas Kedokteran Universitas Indonesia for conducting real time PCR analysis for our samples used in this study.
References
-
Zuk
P.A.,
Zhu
M.,
Ashjian
P.,
De Ugarte
D.A.,
Huang
J.I.,
Mizuno
H.,
Human adipose tissue is a source of multipotent stem cells. Molecular Biology of the Cell.
2002;
13
(12)
:
4279-95
.
View Article PubMed Google Scholar -
Zuk
P.A.,
Zhu
M.,
Mizuno
H.,
Huang
J.,
Futrell
J.W.,
Katz
A.J.,
Multilineage cells from human adipose tissue: implications for cell-based therapies. Tissue Engineering.
2001;
7
(2)
:
211-28
.
View Article PubMed Google Scholar -
Koga
H.,
Muneta
T.,
Nagase
T.,
Nimura
A.,
Ju
Y.J.,
Mochizuki
T.,
Comparison of mesenchymal tissues-derived stem cells for in vivo chondrogenesis: suitable conditions for cell therapy of cartilage defects in rabbit. Cell and Tissue Research.
2008;
333
(2)
:
207-15
.
View Article PubMed Google Scholar -
Remelia
M.,
Rosadi
I.,
Rosliana
I.,
Sobariah
S.,
Karina. Optimized method for isolation of regenerative stromal cells from human lipoaspirates.. The 3rd International Conference of Innovations in Cancer Research and Regenerative Medicine.
2017
.
-
Zhang
X.,
Wei
X.,
Liu
L.,
Marti
G.P.,
Ghanamah
M.S.,
Arshad
M.J.,
Increasing burn severity in mice is associated with delayed mobilization of circulating angiogenic cells. Archives of Surgery (Chicago, Ill.).
2010;
145
(3)
:
259-66
.
View Article PubMed Google Scholar -
Pawitan
J.A.,
Liem
I.K.,
Suryani
D.,
Bustami
A.,
Purwoko
R.Y.,
Simple lipoaspirate washing using a coffee filter. Asian Biomedicine.
2013;
7
(3)
:
333-8
.
-
Van Pham
P.,
Hong-Thien Bui
K.,
Quoc Ngo
D.,
Tan Khuat
L.,
Kim Phan
N.,
Transplantation of nonexpanded adipose stromal vascular fraction and platelet-rich plasma for articular cartilage injury treatment in mice model. Journal of Medical Engineering.
2013;
2013
:
832396
.
View Article PubMed Google Scholar -
Dominici
M.,
Le Blanc
K.,
Mueller
I.,
Slaper-Cortenbach
I.,
Marini
F.,
Krause
D.,
Minimal criteria for defining multipotent mesenchymal stromal cells. The International Society for Cellular Therapy position statement. Cytotherapy.
2006;
8
(4)
:
315-7
.
View Article PubMed Google Scholar -
Maredziak
M.,
Marycz
K.,
Tomaszewski
K.A.,
Kornicka
K.,
Henry
B.M.,
The influence of aging on the regenerative potential of human adipose derived mesenchymal stem cells. Stem Cells International.
2016;
2016
:
2152435
.
View Article PubMed Google Scholar -
Pawitan
J.A.,
Wulandari
D.,
Suryani
D.,
Damayanti
L.,
Purwoko
R.Y.,
Liem
I.K.,
Flow cytometry analysis of adipose tissue-derived stem cells that were cultured in various media. International Journal of Pharm Tech Research.
2013;
5
(3)
:
1301-6
.
-
Hosni Ahmed
H.,
Rashed
L.A.,
Mahfouz
S.,
Elsayed Hussein
R.,
Alkaffas
M.,
Mostafa
S.,
Can mesenchymal stem cells pretreated with platelet-rich plasma modulate tissue remodeling in a rat with burned skin?. Biochemistry and Cell Biology.
2017;
95
(5)
:
537-48
.
View Article PubMed Google Scholar -
Lee
D.E.,
Ayoub
N.,
Agrawal
D.K.,
Mesenchymal stem cells and cutaneous wound healing: novel methods to increase cell delivery and therapeutic efficacy. Stem Cell Research & Therapy.
2016;
7
(1)
:
37
.
View Article PubMed Google Scholar -
Caliari-Oliveira
C.,
Yaochite
J.N.,
Ramalho
L.N.,
Palma
P.V.,
Carlos
D.,
Cunha
F.Q.,
Xenogeneic mesenchymal stromal cells improve wound healing and modulate the immune response in an extensive burn model. Cell Transplantation.
2016;
25
(2)
:
201-15
.
View Article PubMed Google Scholar -
Johnson
K.E.,
Wilgus
T.A.,
Vascular endothelial growth factor and angiogenesis in the regulation of cutaneous wound repair. Advances in Wound Care (New Rochelle, N.Y.).
2014;
3
(10)
:
647-61
.
View Article PubMed Google Scholar -
Muhammad
G.,
Xu
J.,
Bulte
J.W.,
Jablonska
A.,
Walczak
P.,
Janowski
M.,
Transplanted adipose-derived stem cells can be short-lived yet accelerate healing of acid-burn skin wounds: a multimodal imaging study. Scientific Reports.
2017;
7
(1)
:
4644
.
View Article PubMed Google Scholar -
Pham
P. Van,
Bui
K.H.,
Ngo
D.Q.,
Doan
T.T.,
Vu
N.B.,
Truong
N.H.,
Regenerative MedicineSpringer: London; 2015.
View Article Google Scholar -
Peng
C.,
Ma
J.,
Gao
X.,
Tian
P.,
Li
W.,
Zhang
L.,
High glucose induced oxidative stress and apoptosis in cardiac microvascular endothelial cells are regulated by FoxO3a. PLoS One.
2013;
8
(11)
:
e79739
.
View Article PubMed Google Scholar -
Ko
K.I.,
Coimbra
L.S.,
Tian
C.,
Alblowi
J.,
Kayal
R.A.,
Einhorn
T.A.,
Diabetes reduces mesenchymal stem cells in fracture healing through a TNFα-mediated mechanism. Diabetologia.
2015;
58
(3)
:
633-42
.
View Article PubMed Google Scholar -
Jiang
T.,
Xu
G.,
Wang
Q.,
Yang
L.,
Zheng
L.,
Zhao
J.,
In vitro expansion impaired the stemness of early passage mesenchymal stem cells for treatment of cartilage defects. Cell Death & Disease.
2017;
8
(6)
:
e2851
.
View Article PubMed Google Scholar
Comments
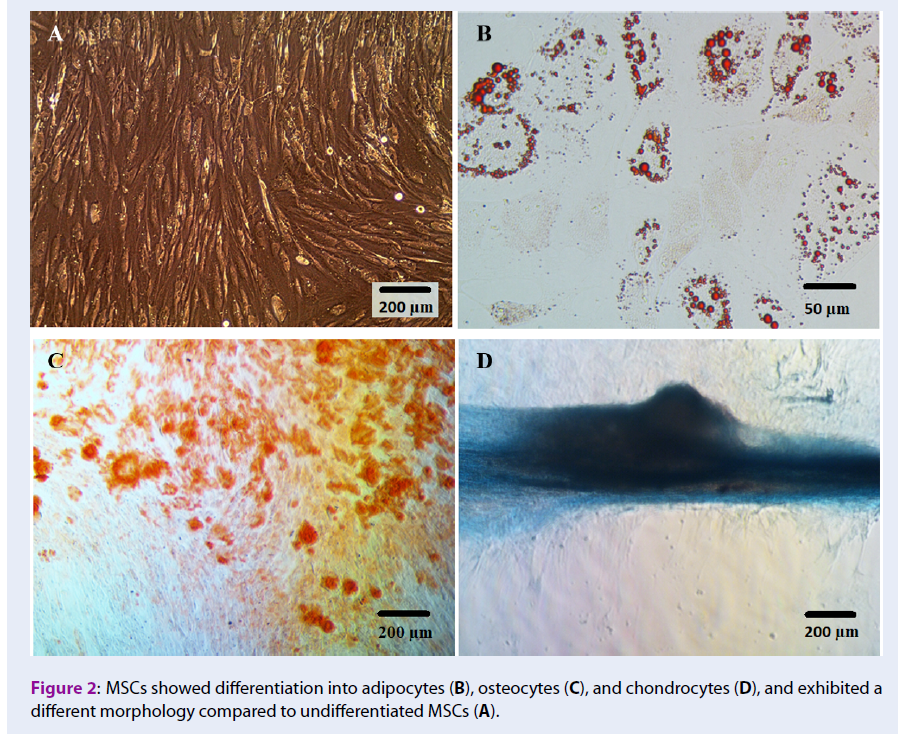
Downloads
Article Details
Volume & Issue : Vol 6 No 10 (2019)
Page No.: 3412-3421
Published on: 2019-10-27
Citations
Copyrights & License

This work is licensed under a Creative Commons Attribution 4.0 International License.
Search Panel
Pubmed
Google Scholar
Pubmed
Google Scholar
Pubmed
Google Scholar
Pubmed
Google Scholar
Pubmed
Google Scholar
Pubmed
Search for this article in:
Google Scholar
Researchgate
- HTML viewed - 5336 times
- Download PDF downloaded - 1061 times
- View Article downloaded - 0 times