Abstract
Introduction: The massive hepatic necrosis of acute liver failure (ALF) results in a sudden loss of hepatic cells. Although most hepatocyte cells of ALF are completely lost, stem cell-derived circulating cells and endogenous progenitor cells rapidly regenerate them. Mesenchymal stem cells (MSCs) have a critical role in the regeneration of liver injury through regulating platelet-derived growth factor (PDGF) and vascular endothelial growth factor (VEGF) levels. However, their fluctuating levels in the healing process and correlation to the decrease of liver function markers remain unclear. The aim of this study was to analyze the effects of MSCs in accelerating liver regeneration of ALF by measuring VEGF and PDGF levels on day 2 and 7, as well as SGPT and SGOT levels, and assessing histopathology appearance.
Methods: Using an ALF rat model, 12 animals were randomly assigned into two groups: umbilical cord (UC)-MSC injection (T1) and vehicle control (Veh). ELISA assay was employed to measure PDGF and VEGF levels, an automatic analyzer was used to assess serum glutamic pyruvic transaminase (SGPT) and serum glutamic oxaloacetic transaminase (SGOT), and hematoxylin and eosin (H&E) staining was used to evaluate morphological appearance.
Results: The study showed an significant (P<0.001) increase of PDGF and VEGF levels on the 2nd day, followed by a decrease on the 7th day, along with a decrease of SGPT and SGOT levels as well as the normality of histology appearance.
Conclusion: In conclusion, administration of MSCs may accelerate liver regeneration of ALF through PDGF and VEGF regulation.
Introduction
Acute liver failure (ALF) is characterized by extensive hepatic necrosis of multiple lobes or acini, and diffuse intra-hepatic infiltration of inflammatory cells without pre-existing liver disease 1. The massive inflammation and hepatocyte necrosis of ALF may lead to rapid hepatocellular dysfunction and multiple organ failure which increase mortality rate 2. Even when the majority of hepatocytes are diminished in ALF, there is still the ability to restore them since hepatocytes are one of the highly regenerative cells. Specifically, the regeneration process is induced by the activation of liver progenitor cells (LPCs) derived from endogenous progenitors (local cells) and or bone marrow-derived circulating cells, particularly mesenchymal stem cells (MSCs) 3. Studies have demonstrated that MSCs have high differentiation potential to specific cells, including primary hepatocytes 4. These facts suggest that MSCs could accelerate the regeneration process of ALF.
MSCs are defined as a plastic-adherent stromal cells with multipotent differentiation capacity and which express various surface markers, including CD73, CD90, CD105, CD44 and CD29, while lacking the expression of CD45, CD34, CD14 or CD11b, CD79a or CD19, and Human Leucocyte Antigen (HLA) class II. MSCs are able to differentiate into chondrocytes, osteocytes and adipocytes 5, including hepatocytes under standard in vitro differentiating conditions 6. On the other hand, MSCs also have immunosuppression capability by suppressing the release of pro-inflammatory molecules which then trigger regeneration via increasing platelet-derived growth factor (PDGF) and vascular endothelial growth factor (VEGF) levels 7. The subsequent improved liver function is marked by normal liver enzymes, serum glutamic pyruvic transaminase (SGPT) and serum glutamic oxaloacetic transaminase (SGOT), and histopathological appearance 8.
Previous studies have reported that VEGF has an important role in enhancing regeneration 9. Moreover, it directs MSC motility to sites of injury via binding co-receptor CD44 to VEGFR-2 in damaged tissues 10. Meanwhile, PDGF also plays a role in the initial inflammatory phase as a chemokine molecule to attract inflammatory cells, including MSCs. Under controlled inflammation, VEGF and PDGF trigger the proliferation phase 1112.
Several studies have demonstrated that the MSCs may migrate to the injured sites, including in ALF, due to their MSC-like leukocyte properties. To reach the target areas, MSCs respond to liver injury-released chemoattractant molecules, such as stromal cell-derived factor 1 (SDF-1), basic fibroblast growth factor (bFGF), and VEGF. In injury areas, MSCs may suppress the inflammation process by releasing several anti-inflammatory cytokines, such as TSG-6, PGE2, IL-1ra, IDO, NO, IL-10 and TGF-β, that alter inflammation to proliferation phase 13. This process may accelerate the regeneration of injured cells in ALF, marked by increasing PDGF and VEGF levels which correlate to normality of liver function. However, the excess of PDGF and VEGF levels can also exert deleterious effects and are associated with increased fibrosis 14. Therefore, controlling the fluctuation of PDGF and VEGF levels by MSCs, particularly in inflammation and proliferation phase, is needed. The aim of this study was to analyze the role of PDGF and VEGF released by MSCs (on days 2 and 7) in ALF that are associated with the improvement of SGOT and SGPT levels, as well as histological appearance.
Methods
Isolation of MSCs
Umbilical cord (UC) derived MSCs (UC-MSCs) were obtained from pregnant single Sprague-Dawley (SD) rats under deep anesthesia and transplanted into an ALF rat model. Briefly, the umbilical cord was cut into pieces after the blood vessels were removed and then transferred to a T25 culture flask containing complete Dulbecco's Modified Eagle's medium (DMEM) (Sigma-Aldrich, Louis St, MO) mixed with 10% Fetal Bovine Serum (FBS) (Gibco™ Invitrogen, NY, USA), and 100 IU/mL penicillin/streptomycin (Sigma-Aldrich). These cells were incubated in a 5% CO2, 37°C incubator and the medium was changed every 3 days. After cells reached 80% confluency, the MSC-like cells were passaged with trypsin. Cells from the 4th passage were used for experiments. This study was approved by the Institutional Review Board of the Ethics Committee of the Medical Department, Sultan Agung Islamic University, in Semarang, Indonesia.
Acute liver failure (ALF) animal model
Twelve male SD rats weighing 200 g (5-weeks of age) were placed in cages at 24°C with 12 h light dark cycle, and fed with water and food. All of the rats were induced by intraperitoneal injection of 0.1 mL/kg carbon tetrachloride (CCl4) (Sigma-Aldrich) dissolved in olive oil (1:1) 2 times per week for 2 weeks to induce ALF in the animals.
Flow cytometric immunophenotyping of UC-MSCs
The immunophenotypes of UC-MSCs were analyzed at fourth passage. UC-MSCs were stained with antibodies conjugated: fluorescein isothiocyanate (FITC)-conjugated CD90, Allophycocyanin (APC)-conjugated CD73, Peridinin Chlorophyll Protein Complex (PerCP)-conjugated CD105 and phycoerythrin (PE)-conjugated Lin monoclonal antibodies for 30 min at 4°C in the dark. The fluorescence intensity of the cells was evaluated through flow cytometry (BD Bioscience, Franklin Lakes, NJ, USA).
In Vitro Differentiation
UC-MSC differentiation potential was determined in order to characterize the isolated cells. These cells were cultured in DMEM medium supplemented with 10% FBS, 10 mmol/L β glycerophospate, 10-7 mol/L/ 0.1 μM dexamethasone, 50 μmol/L ascorbate-2-phosphate (all from Sigma-Aldrich, Louis St, MO), at 37°C and 5% CO2. The fixed cells were stained with 0.2 % Alizarin Red solution (Sigma-Aldrich) to represent calcium deposition (cells used were from the 4th passage).
Administration of MSCs
We isolated MSCs from single rat umbilical cord and transplanted the UC-MSCs into other rats (allogenic transplantation). Experimental rats were randomly assigned into the UC‐MSC injection group (T1, n=6), which received 1 x 106 UC-MSCs dissolved in 1 mL NaCl, or the vehicle control group (Veh, n=6), which received only NaCl; administration was done via lateral tail vein injection.
Histological Analysis
All experimental rats were sacrificed by cervical dislocation on the 7th day after MSC injection. The liver tissue of each animal was fixed in 10% formaldehyde, embedded with paraffin, and sectioned. The histological appearance was observed after hematoxylin & eosin (H&E) staining at high magnification.
Enzyme-linked immunosorbent assay (ELISA)
The blood of rats was harvested via periorbital venous plexus bleeding under general anesthesia on days 0 (pre-treatment), 2 and 7, and the serum was collected by centrifugation at 4°C. We measured PDGF and VEGF concentrations by enzyme-linked immunosorbent assay (ELISA) kits, based on the manufacturer’s instructions (Fine Test, Wuhan, China) and according to a standard curve constructed for each assay. The colorimetric absorbance was recorded at a wavelength of 450 nm.
Liver function enzyme assays
SGOT and SGPT were measured to determine liver functions at days 0 (pre-treatment) and 7. Blood samples were collected from periorbital veins under anesthesia with ketamine + xylazine (100 mg/kg + 5 mg/kg i.m.) (Alfasan, Netherlands). The serum was collected by centrifugation at 4°C. SGOT and SGPT serum levels were measured using an automatic analyzer (BT 3000 PLUS, Italy).
Results
Characteristics of UC-MSCs
After 4 passages, the expression of specific markers of UC-MSCs were evaluated using flow cytometric analysis (Figure 1). The results showed that the isolated cells expressed a MSC-specific marker profile. Indeed, the showed positive expression of CD105 (96.7%), CD73 (99.2%), and CD90 (96.7%), but were negative for Lin (0.03%). Note that the Lin- cell subset serves as a hematopoetic lineage negative marker, according to the International Society of Cellular Therapy (ISCT) Figure 1.
Isolation and osteogenic differentiation of UC-MSCs
UC-MSC isolation was performed based on the plastic adherent capacity under standard culture conditions (37oC, 5% CO2). The isolated cells showed peculiar fibroblast-like (spindle shape) morphology (magnification x10, scale bar 200 µm) (Figure 2A). To determine and verify the multi-lineage differentiation of UC-MSCs, we assessed osteogenic differentiation after 4 passages. The differentiation assay indicated that the multipotency of cultured UC-MSCs was well-maintained which was identified as calcium deposits (red color) by Alizarin red dye (Figure 2B) (magnification x40, scale bar 50 µm).
Histological analysis
To confirm the effects of the treatment groups and that they were conducted in an ALF animal model, the liver tissues of pre-treated animals were stained by H&E (Figure 3A). The result showed that the hepatocytes of pre-treatment group are ballooned, with rounded plasma membrane contours. These indicated that all animal model groups were in ALF condition. The effects of UC-MSCs on liver tissue of animals in the ALF model were evaluated by H&E staining of liver tissue slides. The results showed that there were different morphologies of the hepatocytes in the vehicle treatment group versus the UC-MSC treatment group. The histological features of liver tissues treated with UC-MSCs (Figure 3C) showed normal arrangement of hepatocytes, narrow sinusoid and small hepatic arteries, compared to vehicle group (on the 7th day) (magnification x10). In the vehicle group, there was sinusoidal dilatation and irregular shape of small twigs with hyperchromatic nuclei.
VEGF and PDGF levels
We performed the level of VEGF and PDGF (five replicates per sample). The analysis of PDGF concentration showed that there was significant increase (P<0.05) in treatment group at the 2nd day (343.67±5.21 pg/mL), compared to the vehicle group (290.42±7.61 pg/mL). However, there was significant decrease (P<0.05) in treatment group at 7th day (263.79±3.99 pg/mL), compared to the vehicle control (315.80±5.82 pg/mL). Furthermore, VEGF concentration also showed significant increase (P<0.05) in treatment group at 2nd (110.11±2.41 pg/mL) and 7th day (110.31±6.01 pg/mL). Thus, the optimal concentration was found on the 2nd day Figure 4.
SGPT and SGOT levels
SGPT and SGOT levels were measured to determine the liver functions.The results of the pre-treatment group (day 0) showed high levels of SGPT (>200 U/L) and SGOT (>300 U/L), which indicate that all treated animals were undergoing ALF. The result showed that there was significant decrease (P<0.05) in SGPT (52.40±4.72 U/L) and SGOT (105.80±10.32 U/L) levels on day 7th in the treatment group, as compared to vehicle group (SGPT=128.60±15.93 U/L; SGOT=146.80±10.49 U/L) Figure 5.
Discussion
In recent years, UC-MSCs have been used as a new therapeutic paradigm for a number of diseases in regenerative medicine. Several studies have shown that stem cell based therapy may reduce liver inflammation and improve hepatocyte cell regeneration. Thus, use of UC-MSCs could be a promising strategy for liver injury, including ALF. The massive progression of necrosis of liver cells in ALF may result from a sudden loss of hepatic cells which potentially lead to multiple organ failure and death 11. Therefore, a new treatment approach focusing on liver regeneration would provide great clinical value. To analyze the effects of MSCs on accelerating liver regeneration of ALF, we used carbon tetrachloride (CCl4)-injected Sprague-Dawley rats as an established ALF animal model, according to previous protocols 15. In this study, we injected UC-MSCs into SD rats via i.v. route and then analysing VEGF and PDGF levels on day 2 and 7. We also measured SGOT and SGPT levels, as well as histopathological appearance on day 7.
We found that the PDGF and VEGF levels were significantly increased on the 2nd day (p<0.05). It was presumed that the inflammation process of ALF had been controlled post UC-MSC administration. Under-controlled inflammation processes promote the shifting of inflammation to the proliferation phase, as indicated by release of PDGF and VEGF as potent proliferation molecules (Figure 4B). On the contrary, in the control group, the levels of PDGF and VEGF are much lower due to the fact that the inflammation process is still in progress.
In addition, inflammation releases several inflammatory molecules, particularly serine proteases (SPs), that trigger the proteolytic microenvironment state, leading to degradation of PDGF and VEGF 1216. Specifically, in addition to proliferation, the significant increase of VEGF level at the 2nd day post MSC administration indicated that the MSCs are also actively releasing several chemokine molecule for homing of other circulating MSCs to sites of liver injury 17. This study is in accordance with a previous study which showed the MSC homing process to injured area due to stimulation of specific molecules, namely stromal derived factor-1 (SDF-1), integrin α4, integrin β1 forming very late antigen 4 (VLA-4), and VEGF 14 (Figure 7).
On the other side, VEGF is well-known to play a role as a potent angiogenic factor in hepatocyte regeneration. It has been reported that VEGF expression is related to liver regeneration after chronic liver injury or partial hepatectomy in rats. Moreover, VEGF also produces a significant suppression of hepatocyte apoptosis, indicating that VEGF may reduce mortality rates in ALF 18. Moreover, PDGF may play role in proliferation based on the high expression at day 2. Previous studies have demonstrated that PDGF has an essential role in liver fibrogenesis, potentially acting as a potent mitogen for hepatic stellate cell (HSC) growth 19. This cytokine also plays a role in the initiation phase of hepatocyte regeneration and prevention of hepatocyte apoptosis through Akt or ERK1/2 pathway activation 20.
In sites of injury, MSCs suppress the inflammation process and then promote the proliferation phase. MSCs actively induce the activation of endogenous stem cell to accelerate liver regeneration in ALF; this is known as a paracrine mechanism. This mechanism simultaneously improve the regeneration of hepatocyte cells to an optimal state, then gradually decreases the regeneration 21. This is in line with our study results which show that PDGF and VEGF levels significantly decreased at day 7, in accordance with improvement of SGOT and SGPT levels (clinical markers) (Figure 6). Our study shows that there were also significant decreases in SGOT and SGPT levels at day 7 (p<0.05) (Figure 5). This suggest that the regeneration process of ALF had been completed in the MSC treatment group, as indicated by the normal liver function. The decrease of PDGF and VEGF plays an important role in controlling the normal structure of liver cellular of ALF due to upregulation of their levels, particularly at a proliferation phase correlated with fibrotic disease 22. Specifically, PDGF is the most potent mitogen for activating hepatic stellate cells (HSCs) to trigger extracellular matrix synthesis. This fact is supported by a previous study that demonstrated that PDGF-activated HSCs play a vital role in progression of liver fibrosis 23.
In this study, we also found that the normal appearance of hepatocyte cells in rats from the MSC treatment group at day 7, which indicates a normal arrangement of hepatocytes, such as those from the narrow sinusoid and/or small hepatic arteries (Figure 3). On the contrary, we found mostly sinusoidal dilatation in the vehicle treatment (control) group, as well as an irregular shape of small twigs with hyperchromatic nuclei. These suggest that liver tissues of rats in the control group were still in the proliferation process. This observation shows that MSC treatment group was faster than vehicle control group in accelerating liver regeneration of ALF through PDGF and VEGF regulation. These regulations initially increase the inflammatory phase, reach an optimum point in the proliferation phase, and then gradually decreases to normal levels in the remodeling phase 24.
The limitation of this study is that we did not analyze the in-situ existence (of liver tissue) of MSCs 24 h after administration. Hence, we do not have a clear observation of the MSC homing mechanisms. We also did not analyze other soluble factors that may effect the regeneration process.
Conclusions
We conclude that MSCs can accelerate the regeneration process in ALF by regulating the PDGF and VEGF levels. This is in accordance with the improvement of SGPT and SGOT (liver function) as well as normal arrangement of hepatocyte cells. This study provides new insight on the benefits of MSC administration in most injured tissues, particularly in ALF.
Competing Interests
The author(s) declare that they have no competing interests.
Authors' Contributions
Agung Putra: A, C, D, E, H
Arini Dewi Antari: F, H
Azizah Retno Kustiyah: C
Yulice Soraya Nur Intan: C
Nur Anna C. Sadyah: I
Nugraha Wirawan: G
Samara Astarina: J
Nasrul Zubir: I, H
Delfitri Munir: B, D
Contribution codes : A : Conception and design; B: Analysis and interpretation of the data ; C: Drafting of the article; D: Critical revision of the article for important intellectual content; E: Final approval of the article; F: Provision of study materials or patients; G: Statistical expertise; H: Obtaining of funding; I: Administrative, technical or logistic support; J: Collection and assembly of data.
Acknowledgments
We would like to thanks to Stem Cell and Cancer Research Laboratory, Medical Faculty, Universitas Islam Sultan Agung Semarang for all Facility to finish this research.
Abbreviations
ALF: Acute Liver Failure
APC: Allophycocyanin
bFGF: basic fibroblast growth factor
CCl4: Carbon tetrachloride
CO2: Carbon dioxside
DMEM: Dulbecco’s modified Eagle medium
ELISA: Enzyme-linked immunosorbent assay
FBS: Fetal Bovine Serum
FITC: Fluorescein Isothiocyanate
HE: Hematoxylin-and-eosin
HSCs: Hepatic stellate cells
IDO: Indoleamine 2,3-dioxygenase
IL-10: Interleukin-10
IL-1ra: Interleukin-1 receptor antagonist
ISCT: International Society of Cellular Therapy.
LPC: Liver progenitor cell
MSCs: Mesenchymal Stem Cells
NaCl: Natrium Clorida
NO: Nitrit Oxide
PDGF: Platelet Derived Growth Factor
PE: Phycoerythrin
PGE2: Prostaglandin E2
SD: Sprague-Dawley
SDF-1: Stromal Derived Factor-1
SGOT: Serum Glutamic Oxaloacetic Transaminase
SGPT: Serum Glutamic Piruvic Transaminase
SPs: Serine Proteases
TGF-β: Transforming Growth Factor-beta
TSG-6: Tumor necrosis factor-inducible gene 6
UC-MSCs: Umbilical Cord-Mesenchymal Stem Cells
VEGF: Vascular Endothelial Growth Factor
VEGFR-2: Vascular Endothelial Growth Factor Reseptor-2
References
-
Lee
W. M..
Acute liver failure. Seminars in Respiratory and Critical Care Medicine.
2012;
33
:
36-45
.
View Article PubMed Google Scholar -
Krysko
D. V.,
Denecker
G.,
Festjens
N.,
Gabriels
S.,
Parthoens
E.,
D\'Herde
K..
Macrophages use different internalization mechanisms to clear apoptotic and necrotic cells. Cell Death and Differentiation.
2006;
13
:
2011-22
.
View Article PubMed Google Scholar -
Itoh
T.,
Miyajima
A..
Liver regeneration by stem/progenitor cells. Hepatology (Baltimore, Md.).
2014;
59
:
1617-26
.
View Article PubMed Google Scholar -
Zhou
X.,
Cui
L.,
Zhou
X.,
Yang
Q.,
Wang
L.,
Guo
G..
Induction of hepatocyte-like cells from human umbilical cord-derived mesenchymal stem cells by defined microRNAs. Journal of Cellular and Molecular Medicine.
2017;
21
:
881-93
.
View Article PubMed Google Scholar -
Dominici
M.,
Blanc
K. Le,
Mueller
I.,
Slaper-Cortenbach
I.,
Marini
F.,
Krause
D..
Minimal criteria for defining multipotent mesenchymal stromal cells. The International Society for Cellular Therapy position statement. Cytotherapy.
2006;
8
:
315-7
.
View Article PubMed Google Scholar -
Chivu
M.,
Dima
S. O.,
Stancu
C. I.,
Dobrea
C.,
Uscatescu
V.,
Necula
L. G..
In vitro hepatic differentiation of human bone marrow mesenchymal stem cells under differential exposure to liver-specific factors. Translational Research ; the Journal of Laboratory and Clinical Medicine.
2009;
154
:
122-32
.
View Article PubMed Google Scholar -
Kocabayoglu
P.,
Lade
A.,
Lee
Y. A.,
Dragomir
A. C.,
Sun
X.,
Fiel
M. I..
?-PDGF receptor expressed by hepatic stellate cells regulates fibrosis in murine liver injury, but not carcinogenesis. Journal of Hepatology.
2015;
63
:
141-7
.
View Article PubMed Google Scholar -
Zhou
R.,
Li
Z.,
He
C.,
Li
R.,
Xia
H.,
Li
C..
Human umbilical cord mesenchymal stem cells and derived hepatocyte-like cells exhibit similar therapeutic effects on an acute liver failure mouse model. PLoS One.
2014;
9
:
e104392
.
View Article PubMed Google Scholar -
Schichor
C.,
Birnbaum
T.,
Etminan
N.,
Schnell
O.,
Grau
S.,
Miebach
S..
Vascular endothelial growth factor A contributes to glioma-induced migration of human marrow stromal cells (hMSC). Experimental Neurology.
2006;
199
:
301-10
.
View Article PubMed Google Scholar -
Aldridge
V.,
Garg
A.,
Davies
N.,
Bartlett
D. C.,
Youster
J.,
Beard
H..
Human mesenchymal stem cells are recruited to injured liver in a ?1-integrin and CD44 dependent manner. Hepatology (Baltimore, Md.).
2012;
56
:
1063-73
.
View Article PubMed Google Scholar -
Detry
O.,
Vandermeulen
M.,
Delbouille
M. H.,
Somja
J.,
Bletard
N.,
Briquet
A..
Infusion of mesenchymal stromal cells after deceased liver transplantation: A phase I-II, open-label, clinical study. Journal of Hepatology.
2017;
67
:
47-55
.
View Article PubMed Google Scholar -
Nugraha
A.,
Putra
A..
Tumor necrosis factor-?-activated mesenchymal stem cells accelerate wound healing through vascular endothelial growth factor regulation in rats. Universa Medicina.
2018;
37
:
125-32
.
View Article Google Scholar -
Prockop
D. J.,
Oh
J. Y..
Mesenchymal stem/stromal cells (MSCs): role as guardians of inflammation. Molecular Therapy.
2012;
20
:
14-20
.
View Article PubMed Google Scholar -
Bai
Q.,
An
J.,
Wu
X.,
You
H.,
Ma
H.,
Liu
T..
HBV promotes the proliferation of hepatic stellate cells via the PDGF-B/PDGFR-? signaling pathway in vitro. International Journal of Molecular Medicine.
2012;
30
:
1443-50
.
View Article PubMed Google Scholar -
Meier
Raphael PH,
Mahou
Redouan,
Morel
Philippe,
Meyer
Jeremy,
Montanari
Elisa,
Muller
Yannick D,
Christofilopoulos
Panayiotis,
Wandrey
Christine,
Gonelle-Gispert
Carmen,
Bühler
Leo H.
Microencapsulated human mesenchymal stem cells decrease liver fibrosis in mice. Journal of Hepatology.
2015;
62
:
634-641
.
-
Eming
S. A.,
Krieg
T.,
Davidson
J. M..
Inflammation in wound repair: molecular and cellular mechanisms. The Journal of Investigative Dermatology.
2007;
127
:
514-25
.
View Article PubMed Google Scholar -
De Becker
A.,
Riet
I. V..
Homing and migration of mesenchymal stromal cells: how to improve the efficacy of cell therapy?. World Journal of Stem Cells.
2016;
8
:
73-87
.
View Article Google Scholar -
Tanaka
Y.,
Sohda
T.,
Matsuo
K.,
Anan
A.,
Irie
M.,
Takeyama
Y..
Vascular endothelial growth factor reduces Fas-mediated acute liver injury in mice. Journal of Gastroenterology and Hepatology.
2008;
23
:
e207-11
.
View Article Google Scholar -
Stock
P.,
Monga
D.,
Tan
X.,
Micsenyi
A.,
Loizos
N.,
Monga
S. P..
Platelet-derived growth factor receptor-?: a novel therapeutic target in human hepatocellular cancer. Molecular Cancer Therapeutics.
2007;
6
:
1932-41
.
View Article PubMed Google Scholar -
Matsuo
R.,
Ohkohchi
N.,
Murata
S.,
Ikeda
O.,
Nakano
Y.,
Watanabe
M..
Platelets strongly induce hepatocyte proliferation with IGF-1 and HGF in vitro. The Journal of Surgical Research.
2008;
145
:
279-86
.
View Article PubMed Google Scholar -
Chen
L.,
Xu
Y.,
Zhao
J.,
Zhang
Z.,
Yang
R.,
Xie
J..
Conditioned Medium from Hypoxic Bone MarrowDerived Mesenchymal Stem Cells Enhances Wound Healing in Mice. PLoS One.
2014;
9
:
1-12
.
View Article PubMed Google Scholar -
Choi
J. W.,
Kim
S.,
Kim
T. M.,
Kim
Y. M.,
Seo
H. W.,
Park
T. S..
Basic fibroblast growth factor activates MEK/ERK cell signaling pathway and stimulates the proliferation of chicken primordial germ cells. PLoS One.
2010;
5
:
e12968
.
View Article PubMed Google Scholar -
Liu
T.,
Mu
H.,
Shen
Z.,
Song
Z.,
Chen
X.,
Wang
Y..
Autologous adipose tissue-derived mesenchymal stem cells are involved in rat liver regeneration following repeat partial hepatectomy. Molecular Medicine Reports.
2016;
13
:
2053-9
.
View Article PubMed Google Scholar -
Cheng
B.,
Liu
H. W.,
Fu
X. B.,
Sun
T. Z.,
Sheng
Z. Y..
Recombinant human platelet-derived growth factor enhanced dermal wound healing by a pathway involving ERK and c-fos in diabetic rats. Journal of Dermatological Science.
2007;
45
:
193-201
.
View Article PubMed Google Scholar
Comments
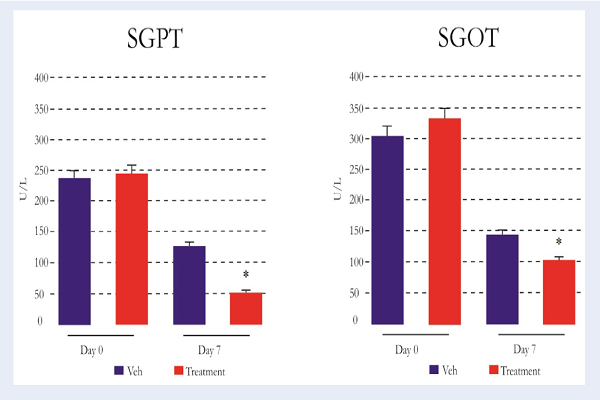
Downloads
Article Details
Volume & Issue : Vol 5 No 11 (2018)
Page No.: 2802-2810
Published on: 2018-11-14
Citations
Copyrights & License

This work is licensed under a Creative Commons Attribution 4.0 International License.
Search Panel
- HTML viewed - 13317 times
- Download PDF downloaded - 2889 times
- View Article downloaded - 0 times