Abstract
Stem cells are not only widely used for regenerative medicine, but are also considered as a useful tool for cancer treatment. For a long time, stem cells have been utilized to renew the immune system for radiation or chemo- therapy treated patients. Recently, stem cells are being engineered to carry therapeutic reagents to target tumor sites. Cancer vaccines based on the knowledge of cancer stem cells have been studied and applied for cancer treatment. In- duced pluripotent stem cells have been used to create active T cells to support cancer immunotherapy. Those are due to the unique characteristics of stem cells, such as immunological tolerance, migration, and tissue reparation. This re- view discusses stem cell applications in transplantation, stem cell-based carriers, induced-pluripotent stem cells, can- cer stem cells, and potential of stem cells engineering to revolutionize cancer treatment.
Introduction
Our bodies contain a pool of stem cells that have the ability to differentiate into any other cell type in the body. Organs and tissues are built up by specialized cells from the pool of stem cells that form shortly after fertilization. Stem cells continue to play a role in repairing damaged tissue and replacing cells that are lost every day. Stem cells are widely defined by two main characteristics: the ability to self-renew (divide in a way that reproduces more identical stem cells) and to differentiate (to turn stem cells into specialized cells that form different organs and tissues).
There are many different kinds of stem cells that exist for different periods of an animal lifetime. For example, embryonic stem cells exist only at the earliest stage of embryo and adult stem cells appear during fetal development and are retained throughout life.
Embryonic stem cells were first identified in mice (Martin, 1981). Embryonic stem cells are pluripotent, meaning they are able to produce all cell types in the body. These cells exist only in earliest stages of embryonic development known as the blastocyst stage. A blastocyst contains an inner cell mass including a clump of around 150 cells that eventually will generate the entire body of the adult animal. When these cells are isolated from the blastocyst and grown in a lab dishes, they can continue dividing indefinitely.
Recently, scientists have discovered how to reprogram normal cells to behave like embryonic stem cells. This is done by re-activating critical genes that define embryonic stem cells to make adult stem cells to revert to an embryonic-like state of pluripotency. These cells are called induced pluripotent stem cells (iPSCs).
The first iPSCs were created from normal cells in the mouse (Takahashi and Yamanaka, 2006). Later in 2007, iPSCs from human skin cells were generated by the same group and created in a number of other laboratories soon afterwards (Park, Lerou, Zhao, Huo, and Daley, 2008; Takahashi et al., 2007; J. Yu et al., 2007; Junying Yu et al., 2007). As the name implies, pluripotent cells are able to generate all other specialized cell types. This way of making iPSCs from adult cells circumvents the need to destroy an embryo from living donor.
Adult stem cells were firstly isolated from bone marrow in mice (Spangrude, Heimfeld, and Weissman, 1988) and later in humans. Some adult stem cell lines are multipotent, in the other words, they have the ability to turn into a defined set of mature cell lines. Some adult stem cell lines are unipotent meaning that they can differentiate into only the cell type of tissue in which they reside. Adult stem cells are found in the tissue of adults, children, and fetuses. However, it is unclear if stem cells are present in all organs. Adult stem cells are rare and difficult to isolate from surrounding tissue.
Adult stem cells are considered as a resource to maintain the turnover rate of cells in the mature tissue. Normally, the body’s cells grow and divide to form new cells, according to body’s demands. When cells become old or damaged, they die as programmed, and new cells will take place. However, when these cells divide out of control and invade into surrounding tissue, they can cause disease, called cancer. Cancer cells do not die as they are supposed to, but they keep proliferating without the body’s demand. These cells may form masses of quickly dividing cells called tumors. Many cancers form solid tumors. However, blood cancer does not form solid tumors.
Cancer is a leading cause of death worldwide, with approximately 14 million new cases and 8.2 million deaths in 2012, according to World Cancer Report 2014. Until now, there are several methods to treat cancer including surgical extraction, chemotherapy, radiation, targeted drug therapy, immunotherapy, and stem cells. Stem cells have contributed significant roles for those approaches of cancer treatment and research. Basing on unique characteristics of stem cells such as self-renew, differentiation, and damage repair, stem cells have been exploited as useful tools in supporting conventional approaches as well as in developing new methods for cancer treatment.
Stem cell transplantation to recover immune system
Stem cell transplantation (SCT) is the procedure that can recover the marrow function for patients who have severe marrow injuries or damaged immune. Stem cell for transplantation can come from bone marrow, peripheral blood, or umbilical cord blood. There are many terms for stem cell transplantation, including bone marrow transplantation, cord blood transplantation, or hematopoietic cell transplantation. These different names are used for the same procedure. There are two common types of transplantation including autologous and allogeneic stem cell transplantation.
With autologous stem cell transplantation, patients use their stem cells. This type of transplantation is used for cancer patients who exposure with a high dose of chemotherapy or radiation therapy. Such high doses of treatment are used to eliminate cancer cells, but can severely damage bone marrow and immune system. Therefore, in order to preserve stem cells, those are collected from bone marrow or blood before treatment, then frozen. Later on, thawed stem cells are re-infused into a patient in order to restore function of the immune system Illerhaus et al., 2006Kessinger et al., 1991 Figure 1 . Because stem cells come from patient’s own, the immune system recovered by stem cell does not attack patient’s tissue. However, those could be contaminated with circulating cancer cells and may increase the risk of rellapse of disease. Furthermore, the recovered immune system could be stronger, but does not have the ability to eliminate the remaining cancer cells since those cancer cells may tolerate to patient’s immune system Igney and Krammer, 2002O’Connell et al., 1999.
Allogeneic stem cell transplantation is typed of treatment that using stem cell from donors that are could be related or unrelated. The compatibility of tissue type, called human leukocyte antigen (HLA), between recipient and donor, is primary criteria in this type of transplantation. Since recipients receive stem cells from another person, it is possible that: immune system of patient reject donated stem cell (host-versusgraft effect), or the donor cells cause immune reaction against tissue of the recipient (graft-versus-host disease [GvHD]). However, before the allogeneic transplant, patients are treated with high doses of chemotherapy or radiation therapy. This treatment eliminates cancer cells and also suppresses the patient’s immune system. Therefore, patient’s immune cells are less able to attack donated stem cell. One of the most important benefits of allogeneic transplant is to generate graft-versus-tumor (GvT) effect. GvT effect is donor immune system recognizing the remaining cancer cells that survived after high doses of chemotherapy, and eliminating them. This effect may help to reduce the risk of disease occurrence. However, GvT effect is often accompanied by GvHD, which is substantially influence mortality after transplantation. In order to reduce GvHD, patients were treated by drug to reduce the ability of donated immune cells to react with patient’s tissue. When the new immune system developed by donated stem cells tolerates to host’s tissue, the patient may reduce or stop using the drug to suppress immune cells. Various factors such as donor selection, stem cell source, immunosuppressive regimen are implemented to balance GvT and GVHD.
Stem cell transplantation supporting high-doses chemotherapy has shown effective in treating a patient with blood cancers. High doses chemotherapy and radiation therapy with SCT is also used for patients with solid tumors such as metastatic renal cell cancer Burk, 2001, advanced ovarian cancer Frickhofen et al., 2006, breast cancer Garcia-Rayo et al., 2001, testicular cancer (Voss, Feldman, and Motzer, 2012) brain tumor (Dunkel and Finlay, 2002), Wilm’s tumor (Kosmas et al., 2010). However, recently studies observed survival SCT patients for 20 years has shown that SCT recipients have a substantial risk of developing solid secondary cancers five years after SCT Inamoto et al., 2015. The secondary cancers include the skin, thyroid, oral cavity, esophagus, liver, brain, bone, and connective tissue. Young age at SCT, chronic GVHD, and prolonged immunosuppressive treatment are supposed to be the risk factors for many types of secondary. Another factor could involve in developing a secondary tumor. For instance, a study on survival SCT blood cancer patients has shown post-high dose sequential radiotherapy associated with risk of secondary malignancy Tarella et al., 2011. While looking toward the improvements to prevent tumor development in survival SCT patients, SCT is still the first line for supporting high doses chemotherapy and radiation therapy in cancer treatment.
Production of cancer-specific T cells from induced pluripotent stem cells
T lymphocytes play an important role in the immune system and are at the core of adaptive immunity to response to specific invaders. In cancer, T-cells search out and destroy the targeted cancer cells. However, the immune system of cancer patients has shown to be modulated so that T cells do not effectively recognize and eliminate cancer cells Baitsch et al., 2011Beurskens et al., 2012Fourcade et al., 2012Oleinika et al.,2013Prado-Garcia et al., 2012Riches et al., 2013Severson et al., 2015. Therefore, studies have been done to offer solutions to produce killer T cells for cancer treatment. Recently, discover of induced pluripotent stem cells (iPSCs) by reprogramming normal cells have triggered new methods to produce cancer cell-specific killer T cells.
There are multiple ways to generate cancer cells- specific T cells through reprogramming techniques. One of those methods is generating iPSCs from mature CD8+ T cells ( Figure 2A ). Mature killer T lymphocytes are reprogrammed into iPSCs by exposing them to Yamanaka reprogramming factors (c-Myc, SOX-2, OCT-4, and KLF-4). These factors are a group of genes that help specialized cells convert into a pluripotent state. The iPSCs are grown in the lab until they reach a large number; then they are induced to differentiate into killer T lymphocytes again. These differentiated killer T cells maintain the same genetic phenotype as the original killer T cells and are fully functional Vizcardo et al., 2013.
Another way to produce specific T cells is generating iPSCs from naïve T cells instead of committed T cells. The first step is to harvest naïve T cells and then expose them to the reprogramming factors. Reprogrammed killer T cells are grown and transduced with recombination receptors for tumor-specific antigens, called chimeric antigen receptor (CAR). Finally, these cells are induced to differentiate into T-lymphocytes with an affinity for the chosen tumor antigen present on cancer cells ( Figure 2B ). This technology was successfully used to create human T cells specific to CD19, a marker expressed by malignant B cells Themeli et al., 2013. These iPSC-derived T cells have been shown to be the same phenotype as peripheral blood T cells and to possess the ability to inhibit tumor growth. Regeneration of T cells from iPSCs is potential to create a mass of therapeutic T cells for cancer treatment in the future.
Stem cells as vectors carrying therapeutic reagents to tumors
In gene therapy for cancer treatment, stem cells are used as vehicles to carry drugs or therapeutic vector viruses to tumors. Stem cells possess two crucial advantages that determine their potential application for gene therapy: tumor tropism and immune-privilege.
Stem cells have intrinsic characteristics to migrate toward the injury sites to support repairing. Cancer is a form of lesion inside the body. Thus, mesenchymal stem cells are postulated to have the ability to migrate towards tumors. In fact, tumors secrete cytokines such as TGF-β, IL-8, EGF, HGF, FGF, and PDGF. These secreted cytokines stimulate MSCs to upregulate che- mokine production and expression of chemokine receptors Escobar et al., 2014, and then making MSCs more able to migrate to the tumor site.
Many studies have used MSCs and neural stem cells(NSCs) to carry suicide enzymes to the tumor site. This approach is expected to avoid systemic toxic effects and leave normal cells intact. Prodrug-activating systems that are commonly used are cytosine deaminase/5-fluorocytosine Mullen et al., 1992Wang et al., 2012, herpes simplex virus thymidine ki- nase/ganciclovir Kokoris and Black, 2002. Once engineered stem cells reach the tumor, the suicide enzymes activate 5-fluorocytosine or ganciclovir to a drug that attacks crucial metabolic pathways in the cells, leading to cell death ( Figure 3 ).Active drugs are able to attack neighboring cancer cells via the gap junction, intracellular communication, and connexins; this process is known as bystander effect Kandouz and Batist, 2010. MSCs- based gene therapy has been used to treat several diseases in animal models including glioblastoma Altaner et al., 2014Altanerova et al., 2012Fei et al., 2012Lee et al., 2009, prostate cancer Cavarretta et al., 2010, melanoma Kucerova et al., 2008, gastrointestinal cancer You et al., 2009, and other malignancies. Along with MSCs, neural stem cells (NSCs) carrying suicide enzyme have shown to reduce tumor volume and to increase survival in mouse model of malignant disease including medulloblastoma Kim et al., 2006, melanoma brain metastases Aboody et al., 2006, glioblastoma Barresi et al., 2003Ito et al., 2010, breast cancer brain metastases Joo et al., 2009, prostate cancer Lee et al., 2013, and breast cancer Yi et al., 2014.
Stem cells are also utilized to delivery immunestimulatory cytokines including IL-12 Duan et al., 2009Eliopoulos et al., 2008Gao et al., 2010, IL-21 Hu et al., 2011, IL-24 Zhang et al., 2013, TNF-a Shahrokhi et al., 2013, and IFN-y Bitsika et al., 2012. TRAIL (tumor necrosis factor related apoptosis induced ligand) Shahrokhi et al., 2013, nanoparticles Gao et al., 2013 and anti-angiogenic factors (Ghaedi et al., 2011).
Cancer stem cells and vaccines for cancers
All cells in a tumor mass are not the same type. A minor subset of cells in the tumor mass are considered to be responsible for facilitating tumor initiation and maintaining tumor growth Chiba et al., 2006Collins et al., 2005Patrawala et al., 2005. These cells are called cancer stem cells or cancer-initiating cells. Cancer stem cells are believed to be transformed from stem cells or progenitor cells, or converted from normal cells Lobo et al., 2007. Cancer stem cells and normal stem cells share many traits, including the ability of self-renew, limited differentiation, enhanced mobility, and proliferation Beier et al., 2007Tinhofer et al., 2014.
Currently the three standard cancer treatment optionsare surgery, radiotherapy and chemotherapy. However, many patients receiving prolonged oncological treatment suffer from complications caused by adverse effects of these treatments. These patients may experience a poor quality of life and will often still relapse post-treatment Goldfarb and Ben-Eliyahu, 2006Nabholtz et al., 1996Valero et al., 1995. The main reason current treatment options fail is thought to be due to cancer stem cells, which cannot be eradicated by traditional treatment modalities. Cancer stem cells divide slowly and are resistant to drugs, therefore, CSCs have the ability to be resistant from radiation and conventional chemotherapy. Due to unlimited proliferation and increased motility, cancer stem cells are thought to give rise to the bulk of the tumor, to promote recurrence, and cause metastases. The cancer stem cell concept therefore implicates new approaches in the treatment of cancer, including specifically targeting cancer stem cells, instead of trying to solely reduce the tumor mass.
Normally the immune system is responsible for retrieving and clearing cancer cells from our body. However, studies have shown that cancer stem cells have the ability to evade the immune system Schneider et al., 2011Wei et al., 2010Wu et al., 2010. Therefore, new cancer treatment therapy to target cancer stem cells by enhancing the immune system is important and necessary.
The process in which the immune system recognizes and kills cancer cells is dependent on the activation of antigen specific T cells by antigen presenting cells including dendritic cells, macrophages, and B cells. Since the discovery of dendritic cells (DCs) by Ralph Steinman in 1973 Steinman and Cohn, 1973, DCs have been shown to perform as professional presenting cells, responsible for triggering an immune response.
Cancer treatments based on dendritic cells enhance the ability of the immune system to recognize CSCs through the presentation of surface antigens. CSCs carry specific-cancer glycans called CSC-markers, also known as CSC-associated antigens. Many efforts to load CSCs specific antigens onto dendritic cells have been performed. In cancer treatment using DCs, therapeutic DCs could be harvested from peripheral blood, or differentiated from peripheral blood-derived monocytes Morse et al., 1997, or cultured bone marrow-derived stem cells Bai et al., 2002. Recently DCs were generated from induced-pluripotent stem cells Senju et al., 2011. DCs are then loaded with cancer antigens by various ways and reinfused into patient ( Figure 4 ). Cancer antigens can be generated from tumor lysates Yu et al., 2004, apoptotic bodies Labarriere et al., 2002, peptides Rosalia et al., 2013, tumor RNAs Kalady et al.,2004, and tumor-derived exosomes Mahaweni et al., 2013. Cancer antigens could be loaded onto DCs by nano-sized carriers including nanoparticles or nanoemulsions Park et al., 2013. Studies on mice have shown DCs primed by CSCs antigens effectively induced immune response to tumor cells and prolonged survival Lu et al.,2015Ning et al., 2012Xu et al., 2009. Treatment with DCs loaded with CSCs-derived antigen induced a tumorspecific immune response stronger than that induced by DCs loaded with normal tumor cells Dillman et al., 2012Jachetti et al., 2013. Recently, clinical study on glioblastoma have shown that when patients were vaccinated with DCs transfected with mRNA derived from patient’s own CSCs, an immune response triggered by vaccination were identified. Compared to untreated patients, progression-free survival was 2.9 times longer in vaccinated patients Vik-Mo et al., 2013.
Conclusion
Current research demonstrates a wide variety of ways engineered stem cells combat diseases like cancer. However, there are still many challenges facing stem cell therapies that remain to be solved. The number of adult stem cells in tissues is limited, and they are difficult to grow in the laboratory. Moreover, it is challenging to gain a sufficient number of adult stem cells types for clinical use. Fortunately, there are new ways to solve some of these problems. For instance, bloodforming stem cells make up a very small percentage of bone marrow. However, large amounts of whole bone marrow can be obtained and administered for transplantation in blood cancers. Another example is the use of adult stem cells, such as mesenchymal stem cells, that can be isolated from an individual and expanded in vitro. However, it is unclear if growing stem cells in the laboratory can induce any mutations that might cause disease later on. Some studies have shown MSCs promote cancer metastasis (Halpern, Kilbarger, and Lynch, 2011; Karnoub et al., 2007; Swamydas, Ricci, Rego, and Dréau, 2013). Another remaining issue is a rejection of transplanted stem cells. Host immune systems reject allogeneic stem cell transplantation and, therefore, require immunosuppressing drugs. Additionally, it can be difficult to find a donor whose human leukocyte antigens closely match the patients’. The recent discovery of reprogramming patient-derived cells has created a breakthrough in this field. Using these cells, known as iPSCs or induced pluripotent stem cells, eliminates the concern for rejection by the patient’s immune system. iPSCs are also easy to grow, proliferate indefinitely and contain the broad potential to form many different cell types. One down side to using iPSC technology is in the process of reprogramming the cells. iPSCs are generated by insertion of genes by viruses into the cells chromosomes. This raises the risk of creating mutations that could transform stem cells into cancer cells. Further studies need to be performed to determine the long-term safety and efficacy of ex vivo expanded stem cells for use in engineering for cancer treatment. It also remains to be determined the optimal dose of engineered stem cells to have the therapeutic effect. There is no doubt that stem cell technology will play a big part in the future of cancer treatment. However, as with any new technology, many issues need to be addressed prior to its widespread use.
Abbreviations
CSCs: Cancer stem cells; DC: dendritic cells; MSCs: Mesenchymal stem cells; SCT: stem cell transplant; iPSCs: induced pluripotent stem cells.
References
-
K.S.
Aboody,
J.
Najbauer,
N.O.
Schmidt,
W.
Yang,
J.K.
Wu,
Y.
Zhuge,
W.
Przylecki,
R.
Carroll,
P.M.
Black,
G.
Perides.
Targeting of melanoma brain metastases using engineered neural stem/progenitor cells. Neuro-oncology.
2006;
8
:
119-126
.
-
C.
Altaner,
V
Altanerova,
K.
Ondicova,
B.
Rychly,
L.
Baciak,
B.
Mravec.
Complete regression of glioblastoma by mesenchymal stem cells mediated prodrug gene therapy simulating clinical therapeutic scenario. International journal of cancer Journal international du cancer.
2014;
134
:
1458-1465
.
-
V.
Altanerova,
M.
Cihova,
M.
Babic,
B.
Rychly,
K.
Ondicova,
B.
Mravec,
C.
Altaner.
Human adipose tissue-derived mesenchymal stem cells expressing yeast cytosinedeaminase:: uracil phosphoribosyltransferase inhibit intracerebral rat glioblastoma. International journal of cancer Journal international du cancer.
2012;
130
:
2455-2463
.
-
L.
Bai,
M.
Feuerer,
P.
Beckhove,
V
Umansky.
Generation of dendritic cells from human bone marrow mononuclear cells: advantages for clinical application in comparison to peripheral blood monocyte derived cells. International journal of oncology.
2002;
20
:
247-253
.
-
L.
Baitsch,
P.
Baumgaertner,
E.
Devevre,
S.K.
Raghav,
A.
Legat,
L.
Barba,
S.
Wieckowski,
H.
Bouzourene,
B.
Deplancke,
P.
Romero.
Exhaustion of tumor-specific CD8(+) T cells in metastases from melanoma patients. The Journal of clinical investigation.
2011;
121
:
2350-2360
.
-
V
Barresi,
S.
Sipione,
G.
Mudo,
E.
Cattaneo,
D.F.
Condorelli.
Transplantation of prodrug-converting neural progenitor cells for brain tumor therapy. Cancer gene therapy.
2003;
10
:
396-402
.
-
D.
Beier,
P.
Hau,
M.
Proescholdt,
A.
Lohmeier,
J.
Wischhusen,
P.J.
Oefner,
L.
Aigner,
A.
Brawanski,
U.
Bogdahn,
C.P.
Beier.
CD133(+) and CD133(-) glioblastoma-derived cancer stem cells show differential growth characteristics and molecular profiles. Cancer research.
2007;
67
:
4010-4015
.
-
F.J.
Beurskens,
M.A.
Lindorfer,
M.
Farooqui,
P.V.
Beum,
P.
Engelberts,
WJ.
Mackus,
P.W
Parren,
R.P.
Taylor.
Exhaustion of cytotoxic effector systems may limit monoclonal antibodybased immunotherapy in cancer patients. Journal of immunology.
2012;
188
:
3532-3541
.
-
V.
Bitsika,
M.G.
Roubelakis,
D.
Zagoura,
O.
Trohatou,
M.
Makridakis,
K.I.
Pappa,
F.C.
Marini,
A.
Vlahou,
N.P.
Anagnou.
Human amniotic fluid-derived mesenchymal stem cells as therapeutic vehicles: a novel approach for the treatment of bladder cancer. Stem cells and development.
2012;
21
:
1097-1111
.
-
R.D.
Burk.
Treatment of renal-cell cancer by transplantation of allogeneic stem cells. The New England journal of medicine.
2001;
344
:
137; author reply 138
.
-
I.T.
Cavarretta,
V.
Altanerova,
M.
Matuskova,
L.
Kucerova,
Z.
Culig,
C.
Altaner.
Adipose tissue-derived mesenchymal stem cells expressing prodrug-converting enzyme inhibit human prostate tumor growth. Molecular therapy : the journal of the American Society of Gene Therapy.
2010;
18
:
223-231
.
-
T.
Chiba,
K.
Kita,
Y.W
Zheng,
H.
Saisho,
A.
Iwama,
H.
Nakauchi,
H.
Taniguchi.
Side population purified from hepatocellular carcinoma cells harbors cancer stem cell-like properties. Hepatology.
2006;
44
:
240-251
.
-
A.T.
Collins,
P.A.
Berry,
C.
Hyde,
M.J.
Stower,
N.J.
Maitland.
Prospective identification of tumorigenic prostate cancer stem cells. Cancer research.
2005;
65
:
10946-10951
.
-
R.O.
Dillman,
A.N.
Cornforth,
C.
Depriest,
E.F.
McClay,
T.T.
Amatruda,
C.
de Leon,
R.E.
Ellis,
C.
Mayorga,
D.
Carbonell,
J.M.
Cubellis.
Tumor stem cell antigens as consolidative active specific immunotherapy: a randomized phase II trial of dendritic cells versus tumor cells in patients with metastatic melanoma. Journal of immunotherapy.
2012;
35
:
641-649
.
-
X.
Duan,
H.
Guan,
Y.
Cao,
E.S.
Kleinerman.
Murine bone marrow-derived mesenchymal stem cells as vehicles for interleukin-12 gene delivery into Ewing sarcoma tumors. Cancer.
2009;
115
:
13-22
.
-
N.
Eliopoulos,
M.
Francois,
M.N.
Boivin,
D.
Martineau,
J.
Galipeau.
Neo-organoid of marrow mesenchymal stromal cells secreting interleukin-12 for breast cancer therapy. Cancer research.
2008;
68
:
4810-4818
.
-
G.
Escobar,
D.
Moi,
A.
Ranghetti,
P.
Ozkal-Baydin,
M.L.
Squadrito,
A.
Kajaste-Rudnitski,
A.
Bondanza,
B.
Gentner,
M.
De Palma,
R.
Mazzieri.
Genetic Engineering of Hematopoiesis for Targeted IFN-alpha Delivery Inhibits Breast Cancer Progression. Science translational medicine.
2014;
6
:
217ra213
.
-
S.
Fei,
X.
Qi,
S.
Kedong,
J.
Guangchun,
L.
Jian,
Q.
Wei.
The antitumor effect of mesenchymal stem cells transduced with a lentiviral vector expressing cytosine deaminase in a rat glioma model. Journal of cancer research and clinical oncology.
2012;
138
:
347-357
.
-
J.
Fourcade,
Z.
Sun,
O.
Pagliano,
P.
Guillaume,
I.F.
Luescher,
C.
Sander,
J.M.
Kirkwood,
D.
Olive,
V.
Kuchroo,
H.M.
Zarour.
CD8(+) T cells specific for tumor antigens can be rendered dysfunctional by the tumor microenvironment through upregulation of the inhibitory receptors BTLA and PD-1. Cancer research.
2012;
72
:
887-896
.
-
N.
Frickhofen,
W.E.
Berdel,
F.
Opri,
R.
Haas,
A.
Schneeweiss,
M.
Sandherr,
W
Kuhn,
D.K.
Hossfeld,
C.
Thomssen,
H.
Heimpel.
Phase I/II trial of multicycle high-dose chemotherapy with peripheral blood stem cell support for treatment of advanced ovarian cancer. Bone marrow transplantation.
2005;
38
:
493-499
.
-
P.
Gao,
Q.
Ding,
Z.
Wu,
H.
Jiang,
Z.
Fang.
Therapeutic potential of human mesenchymal stem cells producing IL-12 in a mouse xenograft model of renal cell carcinoma. Cancer letters.
2010;
290
:
157-166
.
-
Z.
Gao,
L.
Zhang,
J.
Hu,
Y.
Sun.
Mesenchymal stem cells: a potential targeted-delivery vehicle for anti-cancer drug, loaded nanoparticles. Nanomedicine :.
2013;
nanotechnology
:
biology, and medicine 9, 174-184
.
-
S.
Garcia-Rayo,
J.
Perez-Calvo,
S.
Martin-Algarra,
R.
Martinez-Monge,
O.
Fernandez-Hidalgo,
L.
Subira,
M.
Martinez-Aguillo,
J.
Rebollo,
I.
Azinovic,
A.
Brugarolas.
Multiple cycles of dose-intensive chemotherapy with repeated stem cell support as induction treatment in metastatic breast cancer: a feasibility study. Bone marrow transplantation.
2001;
28
:
235-242
.
-
M.
Ghaedi,
M.
Soleimani,
N.M.
Taghvaie,
M.
Sheikhfatollahi,
K.
Azadmanesh,
A.S.
Lotfi,
J.
Wu.
Mesenchymal stem cells as vehicles for targeted delivery of anti-angiogenic protein to solid tumors. The journal of gene medicine.
2011;
13
:
171-180
.
-
Y.
Goldfarb,
S.
Ben-Eliyahu.
Surgery as a risk factor for breast cancer recurrence and metastasis: mediating mechanisms and clinical prophylactic approaches. Breast disease.
2006;
26
:
99-114
.
-
W.
Hu,
J.
Wang,
X.
He,
H.
Zhang,
F.
Yu,
L.
Jiang,
D.
Chen,
J.
Chen,
J.
Dou.
Human umbilical blood mononuclear cell-derived mesenchymal stem cells serve as interleukin-21 gene delivery vehicles for epithelial ovarian cancer therapy in nude mice. Biotechnology and applied biochemistry.
2011;
58
:
397-404
.
-
F.H.
Igney,
P.H.
Krammer.
Immune escape of tumors: apoptosis resistance and tumor counterattack. Journal of leukocyte biology.
2002;
71
:
907-920
.
-
G.
Illerhaus,
R.
Marks,
G.
Ihorst,
R.
Guttenberger,
C.
Ostertag,
G.
Derigs,
N.
Frickhofen,
F.
Feuerhake,
B.
Volk,
J.
Finke.
High-dose chemotherapy with autologous stem-cell transplantation and hyperfractionated radiotherapy as first-line treatment of primary CNS lymphoma. Journal of clinical oncology : official journal of the American Society of Clinical Oncology.
2006;
24
:
3865-3870
.
-
Y.
Inamoto,
N.N.
Shah,
B.N.
Savani,
B.E.
Shaw,
A.A.
Abraham,
I.A.
Ahmed,
G.
Akpek,
Y.
Atsuta,
K.S.
Baker,
G.W.
Basak.
Secondary solid cancer screening following hematopoietic cell transplantation. Bone marrow transplantation.
2015
.
-
S.
Ito,
A.
Natsume,
S.
Shimato,
M.
Ohno,
T.
Kato,
P.
Chansakul,
T.
Wakabayashi,
S.U.
Kim.
Human neural stem cells transduced with IFN-beta and cytosine deaminase genes intensify bystander effect in experimental glioma. Cancer gene therapy.
2010;
17
:
299-306
.
-
E.
Jachetti,
S.
Mazzoleni,
M.
Grioni,
A.
Ricupito,
C.
Brambillasca,
L.
Generoso,
A.
Calcinotto,
M.
Freschi,
A.
Mondino,
R.
Galli.
Prostate cancer stem cells are targets of both innate and adaptive immunity and elicit tumor-specific immune responses. Oncoimmunology.
2013;
2
:
e24520
.
-
K.M.
Joo,
I.H.
Park,
J.Y.
Shin,
J.
Jin,
B.G.
Kang,
M.H.
Kim,
S.J.
Lee,
M.Y.
Jo,
S.U.
Kim,
D.H.
Nam.
Human neural stem cells can target and deliver therapeutic genes to breast cancer brain metastases. Molecular therapy : the journal of the American Society of Gene Therapy.
2009;
17
:
570-575
.
-
M.F.
Kalady,
M.W.
Onaitis,
S.
Emani,
Z.
Abdul-Wahab,
S.K.
Pruitt,
D.S.
Tyler.
Dendritic cells pulsed with pancreatic cancer total tumor RNA generate specific antipancreatic cancer T cells. Journal of gastrointestinal surgery : official journal of the Society for Surgery of the Alimentary Tract.
2004;
8
:
175-181; discussion 181
.
-
M.
Kandouz,
G.
Batist.
Gap junctions and connexins as therapeutic targets in cancer. Expert opinion on therapeutic targets.
2010;
14
:
681-692
.
-
A.
Kessinger,
J.M.
Vose,
P.J.
Bierman,
J.O.
Armitage.
High- dose therapy and autologous peripheral stem cell transplantation for patients with bone marrow metastases and relapsed lymphoma: an alternative to bone marrow purging. Experimental hematology.
1991;
19
:
1013-1016
.
-
S.K.
Kim,
S.U.
Kim,
I.H.
Park,
J.H.
Bang,
K.S.
Aboody,
K.C.
Wang,
B.K.
Cho,
M.
Kim,
L.G.
Menon,
P.M.
Black.
Human neural stem cells target experimental intracranial medulloblastoma and deliver a therapeutic gene leading to tumor regression. Clinical cancer research : an official journal of the American Association for Cancer Research.
2006;
12
:
5550-5556
.
-
M.S.
Kokoris,
M.E.
Black.
Characterization of herpes simplex virus type 1 thymidine kinase mutants engineered for improved ganciclovir or acyclovir activity. Protein science : a publication of the Protein Society.
2002;
11
:
2267-2272
.
-
L.
Kucerova,
M.
Matuskova,
A.
Pastorakova,
S.
Tyciakova,
J.
Jakubikova,
R.
Bohovic,
V.
Altanerova,
C.
Altaner.
Cytosine deaminase expressing human mesenchymal stem cells mediated tumour regression in melanoma bearing mice. The journal of gene medicine.
2008;
10
:
1071-1082
.
-
N.
Labarriere,
L.
Bretaudeau,
N.
Gervois,
M.
Bodinier,
G.
Bougras,
E.
Diez,
F.
Lang,
M.
Gregoire,
F.
Jotereau.
Apoptotic bodyloaded dendritic cells efficiently cross-prime cytotoxic T lymphocytes specific for NA17-A antigen but not for Melan-A/MART-1 antigen. International journal of cancer Journal international du cancer.
2002;
101
:
280-286
.
-
D.H.
Lee,
Y.
Ahn,
S.U.
Kim,
K.C.
Wang,
B.K.
Cho,
J.H.
Phi,
I.H.
Park,
P.M.
Black,
R.S.
Carroll,
J.
Lee.
Targeting rat brainstem glioma using human neural stem cells and human mesenchymal stem cells. Clinical cancer research : an official journal of the American Association for Cancer Research.
2009;
15
:
4925-4934
.
-
H.J.
Lee,
S.W.
Doo,
D.H.
Kim,
Y.J.
Cha,
J.H.
Kim,
Y.S.
Song,
S.U.
Kim.
Cytosine deaminase-expressing human neural stem cells inhibit tumor growth in prostate cancer-bearing mice. Cancer letters.
2013;
335
:
58-65
.
-
N.A.
Lobo,
Y.
Shimono,
D.
Qian,
M.F.
Clarke.
The biology of cancer stem cells. Annual review of cell and developmental biology.
2007;
23
:
675-699
.
-
L.
Lu,
H.
Tao,
A.E.
Chang,
Y.
Hu,
G.
Shu,
Q.
Chen,
M.
Egenti,
J.
Owen,
J.S.
Moyer,
M.E.
Prince.
Cancer stem cell vaccine inhibits metastases of primary tumors and induces humoral immune responses against cancer stem cells. Oncoimmunology.
2015;
4
:
e990767
.
-
N.M.
Mahaweni,
M.E.
Kaijen-Lambers,
J.
Dekkers,
J.G.
Aerts,
J. P.
Hegmans.
Tumour-derived exosomes as antigen delivery carriers in dendritic cell-based immunotherapy for malignant mesothelioma. Journal of extracellular vesicles.
2013;
2
.
-
M.A.
Morse,
L.J.
Zhou,
T.F.
Tedder,
H.K.
Lyerly,
C.
Smith.
Generation of dendritic cells in vitro from peripheral blood mononuclear cells with granulocyte-macrophage-colony-stimulating factor, interleukin- 4, and tumor necrosis factor-alpha for use in cancer immunotherapy. Annals of surgery.
1997;
226
:
6-16
.
-
C.A.
Mullen,
M.
Kilstrup,
R.M.
Blaese.
Transfer of the bacterial gene for cytosine deaminase to mammalian cells confers lethal sensitivity to 5-fluorocytosine: a negative selection system. Proceedings of the National Academy of Sciences of the United States of America.
1992;
89
:
33-37
.
-
J.M.
Nabholtz,
K.
Gelmon,
M.
Bontenbal,
M.
Spielmann,
G.
Catimel,
P.
Conte,
U.
Klaassen,
M.
Namer,
J.
Bonneterre,
P.
Fumoleau.
Multicenter, randomized comparative study of two doses of paclitaxel in patients with metastatic breast cancer. Journal of clinical oncology : official journal of the American Society of Clinical Oncology.
1996;
14
:
1858-1867
.
-
N.
Ning,
Q.
Pan,
F.
Zheng,
S.
Teitz-Tennenbaum,
M.
Egenti,
J.
Yet,
M.
Li,
C.
Ginestier,
M.S.
Wicha,
J.S.
Moyer.
Cancer stem cell vaccination confers significant antitumor immunity. Cancer research.
2012;
72
:
1853-1864
.
-
J.
O’Connell,
M.W.
Bennett,
G.C.
O’Sullivan,
J.K.
Collins,
F.
Shanahan.
Resistance to Fas (APO-1/CD95)-mediated apoptosis and expression of Fas ligand in esophageal cancer: the Fas counterattack. Diseases of the esophagus : official journal of the International Societyfor Diseases of the Esophagus / ISDE.
1999;
12
:
83-89
.
-
K.
Oleinika,
R.J.
Nibbs,
G.J.
Graham,
A.R.
Fraser.
Suppression, subversion and escape: the role of regulatory T cells in cancer progression. Clinical and experimental immunology.
2013;
171
:
36-45
.
-
Y.M.
Park,
S.J.
Lee,
Y.S.
Kim,
M.H.
Lee,
G.S.
Cha,
I.D.
Jung,
T.H.
Kang,
H.D.
Han.
Nanoparticle-Based Vaccine Delivery for Cancer Immunotherapy. Immune network.
2013;
13
:
177-183
.
-
L.
Patrawala,
T.
Calhoun,
R.
Schneider-Broussard,
J.
Zhou,
K.
Claypool,
D.G.
Tang.
Side population is enriched in tumorigenic, stem-like cancer cells, whereas ABCG2+ and ABCG2- cancer cells are similarly tumorigenic. Cancer research.
2005;
65
:
6207-6219
.
-
H.
Prado-Garcia,
S.
Romero-Garcia,
D.
Aguilar-Cazares,
M.
Meneses- Flores,
J.S.
Lopez-Gonzalez.
Tumor-induced CD8+ T-cell dysfunction in lung cancer patients. Clinical & developmental immunology.
2012;
2012
:
741741
.
-
J.C.
Riches,
J.K.
Davies,
F.
McClanahan,
R.
Fatah,
S.
Iqbal,
S.
Agrawal,
A.G.
Ramsay,
J.G.
Gribben.
T cells from CLL patients exhibit features of T-cell exhaustion but retain capacity for cytokine production. Blood.
2013;
121
:
1612-1621
.
-
R.A.
Rosalia,
E.D.
Quakkelaar,
A.
Redeker,
S.
Khan,
M.
Camps,
J.W.
Drijfhout,
A.L.
Silva,
W.
Jiskoot,
T.
van Hall,
P.A.
van Veelen.
Dendritic cells process synthetic long peptides better than whole protein, improving antigen presentation and T-cell activation. European journal of immunology.
2013;
43
:
2554-2565
.
-
T.
Schneider,
H.
Hoffmann,
H.
Dienemann,
P.A.
Schnabel,
A.H.
Enk,
S.
Ring,
K.
Mahnke.
Non-small cell lung cancer induces an immunosuppressive phenotype of dendritic cells in tumor microenvironment by upregulating B7-H3. Journal of thoracic oncology : official publication of the International Association for the Study of Lung Cancer.
2011;
6
:
1162-1168
.
-
S.
Senju,
M.
Haruta,
K.
Matsumura,
Y.
Matsunaga,
S.
Fukushima,
T.
Ikeda,
K.
Takamatsu,
A.
Irie,
Y.
Nishimura.
Generation of dendritic cells and macrophages from human induced pluripotent stem cells aiming at cell therapy. Gene therapy.
2011;
18
:
874-883
.
-
J.J.
Severson,
H.S.
Serracino,
V.
Mateescu,
C.D.
Raeburn,
R.C. Jr.
McIntyre,
S.B.
Sams,
B.R.
Haugen,
J.D.
French.
PD-1+Tim- 3+ CD8+ T Lymphocytes Display Varied Degrees of Functional Exhaustion in Patients with Regionally Metastatic Differentiated Thyroid Cancer. Cancer immunology research.
2015
.
-
S.
Shahrokhi,
S.
Daneshmandi,
F.
Menaa.
Tumor Necrosis Factor-alpha/CD40 Ligand Engineered Mesenchymal Stem Cells Greatly Enhanced the Anti-Tumor Immune Response and Lifespan in Mice. Human gene therapy.
2013
.
-
R.M.
Steinman,
Z.A.
Cohn.
Identification of a novel cell type in peripheral lymphoid organs of mice. I. Morphology, quantitation, tissue distribution. The Journal of experimental medicine.
1973;
137
:
1142-1162
.
-
C.
Tarella,
R.
Passera,
M.
Magni,
F.
Benedetti,
A.
Rossi,
A.
Gueli,
C.
Patti,
G.
Parvis,
F.
Ciceri,
A.
Gallamini.
Risk factors for the development of secondary malignancy after high-dose chemotherapy and autograft, with or without rituximab: a 20-year retrospective followup study in patients with lymphoma. Journal of clinical oncology : official journal of the American Society of Clinical Oncology.
2011;
29
:
814-824
.
-
M.
Themeli,
C.C.
Kloss,
G.
Ciriello,
V.D.
Fedorov,
F.
Perna,
M.
Gonen,
M.
Sadelain.
Generation of tumor-targeted human T lymphocytes from induced pluripotent stem cells for cancer therapy. Nature biotechnology.
2013;
31
:
928-933
.
-
I.
Tinhofer,
M.
Saki,
F.
Niehr,
U.
Keilholz,
V.
Budach.
Cancer stem cell characteristics of circulating tumor cells. International journal of radiation biology.
2014
.
-
V
Valero,
R.S.
Walters,
R.L.
Theriault,
L.
Esparza,
G.
Fraschini,
G.A.
Fonseca,
R.E.
Bellet,
A.U.
Buzdar,
G.N.
Hortobagyi.
Phase II trial of docetaxel: a new, highly effective antineoplastic agent in the management of patients with anthracyclineresistant metastatic breast cancer. Journal of clinical oncology : official journal of the American Society of Clinical Oncology.
1995;
13
:
2886-2894
.
-
E.O.
Vik-Mo,
M.
Nyakas,
B.V.
Mikkelsen,
M.C.
Moe,
P.
Due-Tonnesen,
E.M.
Suso,
S.
Saeboe-Larssen,
C.
Sandberg,
J.E.
Brinchmann,
E.
Helseth.
Therapeutic vaccination against autologous cancer stem cells with mRNA-transfected dendritic cells in patients with glioblastoma. Cancer.
2013;
immunology
:
immunotherapy : CII 62, 1499-1509
.
-
R.
Vizcardo,
K.
Masuda,
D.
Yamada,
T.
Ikawa,
K.
Shimizu,
S.
Fujii,
H.
Koseki,
H.
Kawamoto.
Regeneration of human tumor antigen-specific T cells from iPSCs derived from mature CD8(+) T cells. Cell stem cell.
2013;
12
:
31-36
.
-
J.
Wang,
S.
Sklenak,
A.
Liu,
K.
Felczak,
Y.
Wu,
Y.
Li,
H.
Yan.
Role of glutamate 64 in the activation of the prodrug 5- fluorocytosine by yeast cytosine deaminase. Biochemistry.
2012;
51
:
475-486
.
-
J.
Wei,
J.
Barr,
L.Y.
Kong,
Y.
Wang,
A.
Wu,
A.K.
Sharma,
J.
Gumin,
V
Henry,
R.
Sawaya.
Glioma-associated cancer-initiating cells induce immunosuppression. Clinical cancer research : an official journal of the American Association for Cancer Research.
2010;
16
:
461-473
.
-
A.
Wu,
J.
Wei,
L.Y.
Kong,
Y.
Wang,
W.
Priebe,
W
Qiao,
A.B.
Heimberger.
Glioma cancer stem cells induce immunosuppressive macrophages/microglia. Neuro-oncology.
2010;
12
:
1113-1125
.
-
Q.
Xu,
G.
Liu,
X.
Yuan,
M.
Xu,
H.
Wang,
J.
Ji,
B.
Konda,
K.L.
Black,
J.S.
Yu.
Antigen-specific T-cell response from dendritic cell vaccination using cancer stem-like cell-associated antigens. Stem cells.
2009;
27
:
1734-1740
.
-
B.R.
Yi,
K.A.
Hwang,
K.S.
Aboody,
E.B.
Jeung,
S.U.
Kim,
K.C.
Choi.
Selective antitumor effect of neural stem cells expressing cytosine deaminase and interferon-beta against ductal breast cancer cells in cellular and xenograft models. Stem cell research.
2014;
12
:
36-48
.
-
M.H.
You,
W.J.
Kim,
W.
Shim,
S.R.
Lee,
G.
Lee,
S.
Choi,
D.Y.
Kim,
Y.M.
Kim,
H.
Kim,
S.U.
Han.
Cytosine deaminaseproducing human mesenchymal stem cells mediate an antitumor effect in a mouse xenograft model. Journal of gastroenterology and hepatology.
2009;
24
:
1393-1400
.
-
J.S.
Yu,
G.
Liu,
H.
Ying,
WH.
Yong,
K.L.
Black,
C.J.
Wheeler.
Vaccination with tumor lysate-pulsed dendritic cells elicits antigen-specific, cytotoxic T-cells in patients with malignant glioma. Cancer research.
2004;
64
:
4973-4979
.
-
X.
Zhang,
L.
Zhang,
W
Xu,
S.
Ye,
W
Zhu,
Y.
Yan,
W.
Li,
M.
Wang.
Experimental therapy for lung cancer: umbilical cord-derived mesenchymal stem cell-mediated interleukin-24 delivery. Current cancer drug targets.
2013;
13
:
92-102
.
Comments
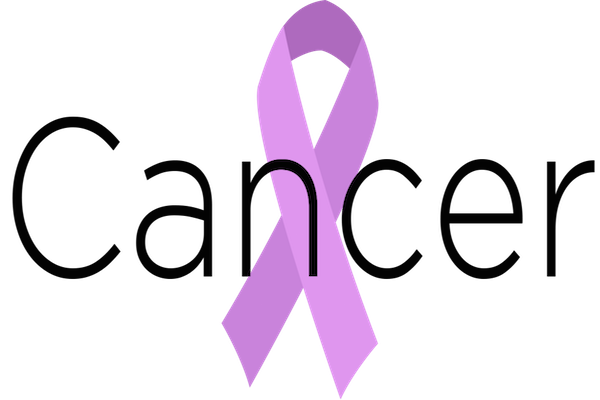
Downloads
Article Details
Volume & Issue : Vol 2 No 06 (2015)
Page No.: 279-289
Published on: 2015-06-12
Citations
Copyrights & License

This work is licensed under a Creative Commons Attribution 4.0 International License.
Search Panel
- HTML viewed - 14132 times
- Download PDF downloaded - 1900 times
- View Article downloaded - 10 times