Abstract
Introduction: This study evaluates the effects of simvastatin on the liver, in a mouse model of DMBA-induced breast cancer, with regards to histopathological, biochemical and antioxidant features.
Methods: Mice were randomly divided into two groups: A (control group) and B (mammary tumor group); the latter group received DMBA (50 mg/kg) by oral gavage once a week for 4 consecutive weeks. Animals positive for breast cancer tumors were randomly divided into 3 subgroups: 1) no treatment group (D1), 2) mice that received simvastatin (80 mg/kg) per os (P.O.) daily for 4 consecutive weeks (D2), and 3) mice that received tamoxifen (50 mg/kg) P.O. daily for 4 consecutive weeks (D3).
Results: Administration of simvastatin to D2 mice resulted in significantly higher superoxide dismutase (SOD) activity as well as glutathione peroxidase (GPx) activity and total antioxidant capacity (TAC), and accompanied by reduced malondialdehyde (MDA) content in liver as compared to D1 group. Tamoxifen significantly increased liver glutathione (GSH) content as compared to D1 mice. Moreover, MDA levels in liver of mice treated with tamoxifen were significantly lower than in the D1 group. Mice in the D1 group showed significantly increased levels of alkaline phosphatase (ALP), aspartate transaminase (AST), and gamma-glutamyl transferase (GGT) in liver tissues; these levels were significantly reduced by simvastatin administration. Moreover, tamoxifen decreased ALP and AST activities. Histopathological examination of liver sections from mice in the D1 group showed severe deteriorative changes. The extent and severity of changes in D2 and D3 groups were almost the same and milder than D1 group.
Conclusion: In conclusion, simvastatin appears to have a hepatoprotective role in mice with DMBA-induced breast cancer, due partly to its antioxidant properties.
Introduction
Statins are routinely prescribed as lipid-lowering drugs Gazzerro et al., 2012 based on their ability for competitive inhibition of 3-hydroxy-3-methyl-glutaryl-coenzyme A (HMG-CoA) reductase activity in liver. Mevalonate, the product of this enzyme, plays an important role not only in cholesterol synthesis but also in production of isoprenoids Stancu and Sima, 2001. In addition to desirable effects on lipid metabolism in liver, statin administration has been associated with beneficial effects in different liver diseases. In fact, statins are shown to reduce the risk of progressive liver fibrosis and protect the liver against infections and ischemia-reperfusion injury. Moreover, new evidence show that statins improve response to interferon-based anti-hepatitis C virus therapy and have favorable effects on hepatocellular carcinoma Bosch and Forns, 2015Pradelli et al., 2013Rao and Pandya, 2011. In general, statins are recognized as well-tolerated drugs which have an excellent safety profile without noticeable adverse side effects after chronic use. However, intolerance to statins may occur in some patients; these include mostly myopathy and alteration of hepatic enzymes which represent liver toxicity Arca and Pigna, 2011Hu et al., 2012.
Transaminitis or transient elevated levels of serum aminotransferase enzymes (in particular, alanine transaminase (ALT) and aspartate transaminase (AST)), without any overt symptoms of hepatotoxicity, is the most frequent type of statin-associated hepatic adverse effects Arca and Pigna, 2011. Some evidence suggest that this may be due to genetic variations in statin metabolism Kalantari and Naghipour, 2014Mulder et al., 2001. Although this elevation may be considered physiological rather than pathological, in rare circumstances statin-induced transaminitis is associated with liver damage Arca and Pigna, 2011Armitage, 2007. The incidence of transaminitis is related directly to statin dose and has been reported to occur in less than 1% of patients receiving low to moderate doses Calderon et al., 2010. Since transaminitis can also occur with the use of other lipid-lowering agents, such as fibrates, resins, niacin and ezetimibe, it may be considered as a hepatic response to lipid-lowering effects of the drugs Armitage, 2007. Taken together, severe liver injury in association with statin use is rare when compared to the magnitude of their use worldwide Arca and Pigna, 2011. Nonetheless, liver enzyme monitoring is recommended during statin therapy Leaver et al., 2009.
Breast cancer is the most common cancer in females and one that many researchers have studied using various chemical-induced models of breast cancer. Of the chemical agents, 7, 12-dimethylbenz(a)anthracene (DMBA) is a potent carcinogen that is used extensively as a prototype agent for induction of mammary tumors in laboratory animals Izzotti et al., 1999. Although liver is not known as a target organ in DMBA-induced carcinogenesis, metabolic activation and detoxification of DMBA takes place in liver cells and during that time, reactive oxygen species (ROS) and DMBA-DNA adducts are formed in hepatocytes Arulkumaran et al., 2007Ip and Lisk, 1997. The ROS generated can react with DNA, lipids and proteins, and exert serious damage Arulkumaran et al., 2007. Consequently, DMBA can induce hepatotoxicity and may exert deleterious effects on hepatocytes Kumar et al., 2014.
Tamoxifen is a common medication for estrogen receptor positive (ER+) breast cancer. In general, it is highly effective and relatively safe. However, like many other drugs, different adverse effects may occur in breast cancer patients taking this medication, including thromboembolic and cardiovascular events, uterine abnormalities, and cataracts Nelson et al., 2013Pan et al., 2016. Additionally, hepatotoxic effects such as fatty liver and hepatocellular damage may be associated with tamoxifen therapy Ching et al., 1992Pan et al., 2016.
The possible effects of statin therapy on liver function and structure in breast cancer patients has not been clarified. In a very recent meta-analysis by Liu et al. (2017) it was clearly shown that lipophilic statins have a strong protective function in breast cancer patients Liu et al., 2017. Although the study showed this promising effect in patients with less than 4 years of follow-up, the finding is clinically valuable and may positively affect the use of statins in breast cancer patients. Considering the fact that both DMBA and tamoxifen may be associated with induction of liver injury, the present study aims to evaluate plausible effects of simvastatin therapy (in comparison with tamoxifen) on liver in a mouse model of DMBA-induced breast cancer, with regards to histopathological, biochemical and antioxidant features of the liver.
Materials-Methods
Animals and experimental design
The study was carried out on female albino mice, initially weighing 23 ± 0.91g and aged 21 days. The mice were purchased from the Comparative and Experimental Medical Center Institute of Preventive Medicine, Shiraz, Iran. They were kept under standard conditions with relative humidity (60%), room temperature (24±4°C) and a 12/12h light dark cycle with free access to commercial pelleted feed and tap water. The experimental protocol was approved by the Institutional Animal Ethical Committee and was conducted in accordance with the Guidelines for the Care and Use of Laboratory Animals, prepared by Shiraz University.
After a week of acclimatization, mice were randomly divided into two groups. Group A (control group; n=7) only received sesame oil as a vehicle by gastric intubation; Group B (mammary tumor group; n=35) received DMBA (50 mg/kg) dissolved in sesame oil once a week by oral route for 4 consecutive weeks beginning at 5 weeks of age. The method for tumor induction was adapted from Currier et al. (2005). Confirmation of tumor induction was made by palpation at 4 weeks after DMBA treatment up to the end of the experiment Currier et al., 2005. When tumor size reached 0.5 cm in the largest dimension, animals (n=21) were randomly divided into 3 subgroups with each containing 7 mice. Subgroup 1 received no specific treatment and represented the DMBA-treated control group (D1), subgroup 2 received 80 mg/kg simvastatin suspended in distilled water by gastric intubation daily for 4 consecutive weeks (D2), and subgroup 3 received 50 mg/kg tamoxifen suspended in distilled water by gastric intubation daily for 4 consecutive weeks (D3). The dosage regimens for tamoxifen were based on a study of Robinson et al. (1989) Robinson et al., 1989. At the end of the experiment, all animals were sacrificed under deep anesthesia and samples from liver were removed immediately. A part of the liver samples was rinsed with ice-cold normal saline and kept in -20°C until further use; another part was kept in buffered formalin for histopathological evaluation.
Preparation of liver tissue homogenate
One hundred mg of liver tissue sample was homogenized on ice in PBS (pH=7.8) by using an ultrasonic tissue homogenizer, and then centrifuged at 12000g for 20 min at 4°C. The supernatant was gently removed for further evaluation and kept at -20°C.
Evaluation of antioxidant/oxidant status of liver tissue homogenate
Total antioxidant capacity (TAC) was measured by the method of Benzie et al. (1996) based on the ferric to ferrous ion reduction and formation of colored ferrous-tripyridyltriazine complex which was measured at 593 nm Benzie and Strain, 1996. It should be mentioned that total protein assay of homogenate was performed by the Lowry method. Superoxide dismutase (SOD) activity was assayed by using a commercial kit (ZellBio, Ulm, Germany). The kit method was based on the fact that SOD enzyme uses superoxide anion as substrate for conversion to hydrogen peroxide and oxygen; the product is a chromogen which is measured at 420 nm.
The catalase (CAT) activity assay was based on the decomposition reaction of hydrogen peroxide (H2O2) as a substrate to water and oxygen by using an appropriate kit prepared by ZellBio, Inc. (Ulm, Germany). The H2O2 produces a product which can be measured at 405 nm and is proportional to the CAT activity.
Glutathione peroxidase (GPx) activity was assayed by using a commercial kit (Randox, UK) based on the oxidation reaction of glutathione (GSH) in the presence of Cumene hydroperoxide by GPx. Oxidized glutathione (GSSG) was immediately converted to the reduced form with concomitant oxidation of NADPH to NADP+. The decrease in absorbance at 340 nm was proportional to GPx activity. Reduced GSH concentration was measured according to reaction of 5,5'-dithiobis(2-nitrobenzoic acid) (DTNB) and GSH, and the generation of yellow compound 2-nitro-5-thiobenzoic acid. Finally, GSH concentration was measured at 412 nm.
Thiobarbituric acid-reactive substances (TBARS) for evaluation of malondialdehyde (MDA) content in liver tissue homogenate were measured spectrophotometrically at 535 nm, as described previously Ohkawa et al., 1979, and calculated according to the molar absorption coefficient of 1.56×105 M-1 cm-1.
Assay of liver function enzymes in liver tissue homogenate
The activity of liver function enzymes including AST, ALT, alkaline phosphatase (ALP), and gamma-glutamyl transferase (GGT) were assayed in liver tissue homogenate by using commercial kits (Pars Azmoon, Tehran, Iran).
Histopathological evaluation of liver tissue
After formalin fixation and routine histological processes, paraffin blocks were made from liver tissues and 5µm-thick sections were stained with hematoxylin and eosin (H&E) method, and blindly evaluated for histopathological changes under light microscope.
Statistical analysis
Data were presented as mean ± standard deviation (SD). Data analysis was performed by one-way ANOVA method followed by Tukey's multiple comparison test as the post hoc (SPSS 11.5 software). P<0.05 was considered as the level of significance.
Results
Antioxidant/oxidant status of liver tissue
Changes in the activity of antioxidant enzymes (including SOD, CAT and GPx), as well as GSH and MDA levels and TAC of liver tissue, in the different groups are summarized in Table 1 . A significant increase in liver MDA content was observed in mice of D1 group as compared to controls (p<0.001). Administration of simvastatin to D2 mice resulted in significantly higher SOD activity (p<0.001), GPx activity (p=0.005) and TAC (p=0.002), and significantly reduced MDA content (p<0.001), as compared to the D1 group. Tamoxifen (D3 group) significantly increased liver GSH content as compared to D1 mice (p=0.015). Moreover, MDA levels in liver of mice treated with tamoxifen were significantly lower than D1 group (p<0.001).
Activity of liver function enzymes
Figure 1-4 illustrate the activity of liver function enzymes in the different groups. Mice in the D1 group showed significantly increased ALP levels as compared to all other groups (p<0.001 for all comparisons). Mice in the D1 group had a significantly higher AST level as compared to controls (p=0.005). Moreover, the administration of simvastatin but not tamoxifen resulted in a significant decrease in the activity of AST as compared to the D1 group (p=0.03).
ALT activity in liver tissues of animals in the various groups were approximately the same (p>0.05). GGT activity in the D1 group significantly increased as compared to control mice (p<0.001); again, administration of simvastatin but not tamoxifen significantly reduced this parameter as compared to D1 mice (p<0.001).
Histopathological changes of liver tissue
Histopathological examination of liver sections from mice in D1 group showed severe necrosis of hepatocytes accompanied by moderate hepatocyte vacuolar degeneration, severe infiltration of inflammatory cells around the portal vein, and moderate sinusoidal dilatation ( Figure 5A Figure 5B ). Furthermore, anaplastic epithelial cells resembling bile duct cells but not hepatocytes (cholangiocellular carcinoma) were observed in one sample ( Figure 5C ). Mice of D2 group that were treated with simvastatin showed mild infiltration of mononuclear inflammatory cells in and around the central vein. Moreover, a mild vacuolar change in hepatocytes was observed ( Figure 5D ). In tamoxifen-treated mice of the D3 group, liver sections showed moderate sinusoidal dilatation. Mild cytoplasmic vacuolation of hepatocytes was also present ( Figure 5E ; Figure 5F ). The extent and severity of changes in the D2 and D3 groups were almost the same.
Discussion
Statins are frequently prescribed for the treatment of hypercholesterolemia and, recently, there has been evolving evidence about diverse promising properties of these agents in breast cancer patients Liu et al., 2017. The effects of statins on liver structure and function have been controversial. For instance, some concerns have been raised in statin users regarding the asymptomatic rise in plasma transaminase concentrations, which may be related due to liver injury Björnsson, 2017Charles et al., 2005. On the contrary, some reports have shown the positive effects of statins in liver diseases Bosch and Forns, 2015Rao and Pandya, 2011.
The present study was aimed to investigate the possible effects of a lipophilic statin (simvastatin) on liver in a mice model of breast cancer and to compare it with a commonly used agent in this disease (tamoxifen). As the first finding of our study, we observed that simvastatin administration in mice with breast cancer is associated with an appreciable improvement in oxidative stress parameters of liver. There was liver deterioration due to DMBA administration, accompanied by a significant reduction in function/activity of liver enzymes (except for ALT which remained statistically the same among all groups).
Biotransformation of DMBA takes place in the liver by cytochrome P450 enzymes which results in generation of diol epoxides and ROS Dakrory et al., 2015. Moreover, DMBA binds firmly to cellular membranes and induces peroxidation of polyunsaturated fatty acids Khyade, 2017. In our study, we consistently observed increased MDA levels in the liver of mice in the D1 group. It is worth to mention that a trivial and insignificant increase in GPx and TAC occurred in D1 group as compared to control mice which may be related to the obvious and statistically significant (more than two times) increase in MDA level of D1 group due to DMBA administration that was not compensated by the slight increase in GPx and TAC. Peroxidation of lipids in cellular membranes is associated with cellular injury and can describe the increased levels of liver function enzymes in the D2 group.
The positive effects of simvastatin on oxidative stress parameters were more pronounced than tamoxifen. Simvastatin was also more effective in reducing GGT than tamoxifen. Antioxidant properties of simvastatin in liver have been previously reported. In a study by Habeos et al. (2008), the authors showed that simvastatin protects rat liver cells against oxidative stress by activating Keap1/Nrf2 signaling pathway Habeos et al., 2008. On the other hand, oxidative stress is known to be involved in different liver injuries including alcohol-induced liver damage Cederbaum et al., 2009 and pathogenesis of hepatitis C virus Choi and Ou, 2006. Therefore, it seems that the antioxidant properties of simvastatin may be involved in protecting the liver from DMBA-induced injury, which was reflected in a better profile of liver function enzyme activity of mice treated with simvastatin. It should be mentioned that since we assayed enzyme activity in liver tissue (not serum), changes in these parameters are directly related to liver status not other organs that also can produce these enzymes.
The results of histopathological evaluation of liver structure also support the ameliorative effect of simvastatin in DMBA-induced liver injury. As previously stated, our findings revealed detrimental histopathological changes, including severe necrosis, infiltration of inflammatory cells, and sinusoidal dilatation in DMBA-treated mice. These results are in accordance with the report of Dakrory et al. (2015) who observed many pathological alterations induced by DMBA in rat liver, including focal necrotic areas of hepatocytes infiltrated with mononuclear cells, congestion of the hepatoportal blood vessel, and leukocyte infiltration Dakrory et al., 2015.
In the present study, simvastatin treatment showed signs of protection against DMBA hepatotoxicity which was almost similar to that of tamoxifen. Apart from antioxidant effects, it has been reported that simvastatin is capable of decreasing intrahepatic vascular resistance and improving liver perfusion Wang et al., 2013; whether this possible mechanism is involved in the hepatoprotective effects of simvastatin in liver injury due to DMBA needs to be confirmed.
Conclusion
In conclusion, simvastatin appears to have a hepatoprotective role in mice with DMBA-induced breast cancer, which is at least partly due to its antioxidant properties.
Abbreviations
ALP: alkaline phosphatase
ALT: alanine aminotransferase
AST: aspartate aminotransferase
CAT: catalase
DMBA: 7, 12-dimethylbenz(a)anthracene
ER+: estrogen receptor positive
GGT: gamma-glutamyl transferase
GPx: glutathione peroxidase
GSH: glutathione
GSSG: oxidized glutathione
HMG-CoA: 3-hydroxy-3-methyl-glutarylcoenzyme A
H2O2: Hydrogen peroxide
MDA: malondialdehyde
ROS: reactive oxygen species
SD: standard deviation
SOD: super oxide dismutase
TAC: total antioxidant capacity
TBARS: thiobarbituric acid-reactive substances
Author Contribution
T. Shomali (corresponding author) and M. Ashrafi substantial contributions to the conception or design of the work and drafting the work or revising it critically for important intellectual content and agreement to be accountable for all aspects of the work in ensuring that questions related to the accuracy or integrity of any part of the work are appropriately investigated and resolved; B. Karimi and M. Sabahi acquisition, analysis, or interpretation of data for the work, all authors made final approval of the version to be published.
References
-
M.
Arca,
G.
Pigna.
Treating statin-intolerant patients. Diabetes. Metabolic Syndrome and Obesity.
2011;
4
.
View Article PubMed Google Scholar -
J.
Armitage.
The safety of statins in clinical practice. Lancet.
2007;
370(9601)
:
1781-1790
.
View Article PubMed Google Scholar -
S.
Arulkumaran,
V. R.
Ramprasath,
P.
Shanthi,
P.
Sachdanandam.
Alteration of DMBA-induced oxidative stress by additive action of a modified indigenous preparation—Kalpaamruthaa. Chemico-Biological Interactions.
2007;
167(2)
:
99-106
.
View Article PubMed Google Scholar -
I. F.
Benzie,
J. J.
Strain.
The ferric reducing ability of plasma (FRAP) as a measure of “antioxidant power”: The FRAP assay. Analytical Biochemistry.
1996;
239(1)
:
70-76
.
View Article PubMed Google Scholar -
E. S.
Björnsson.
Hepatotoxicity of statins and other lipid‐lowering agents. Liver International.
2017;
37(2)
:
173-178
.
-
J.
Bosch,
X.
Forns.
Therapy. Statins and liver disease: From concern to ‘wonder’ drugs?. Nature Reviews. Gastroenterology & Hepatology.
2015;
12(6)
:
320-321
.
-
R. M.
Calderon,
L. X.
Cubeddu,
R. B.
Goldberg,
E. R.
Schiff.
Statins in the treatment of dyslipidemia in the presence of elevated liver aminotransferase levels: A therapeutic dilemma. Mayo Clinic Proceedings.
2010;
85(4)
:
349-356
.
View Article PubMed Google Scholar -
E. C.
Charles,
K. L.
Olson,
B. G.
Sandhoff,
D. L.
McClure,
J. A.
Merenich.
Evaluation of cases of severe statin-related transaminitis within a large health maintenance organization. The American Journal of Medicine.
2005;
118(6)
:
618-624
.
View Article PubMed Google Scholar -
A. I.
Cederbaum,
Y.
Lu,
D.
Wu.
Role of oxidative stress in alcohol-induced liver injury. Archives of Toxicology.
2009;
83(6)
:
519-548
.
View Article PubMed Google Scholar -
C. K.
Ching,
P. G.
Smith,
R. G.
Long.
Tamoxifen-associated hepatocellular damage and agranulocytosis. Lancet.
1992;
339(8798)
:
940
.
View Article PubMed Google Scholar -
J.
Choi,
J. H.
Ou.
Mechanisms of liver injury. III. Oxidative stress in the pathogenesis of hepatitis C virus. American Journal of Physiology. Gastrointestinal and Liver Physiology.
2006;
290(5)
:
G847-G851
.
-
N.
Currier,
S. E.
Solomon,
E. G.
Demicco,
D. L.
Chang,
M.
Farago,
H.
Ying,
D. C.
Seldin.
Oncogenic signaling pathways activated in DMBA-induced mouse mammary tumors. Toxicologic Pathology.
2005;
33(6)
:
726-737
.
View Article PubMed Google Scholar -
A. I.
Dakrory,
S. R.
Fahmy,
A. M.
Soliman,
A. S.
Mohamed,
S. A.
Amer.
Protective and curative effects of the sea cucumber Holothuria atra extract against DMBA-induced hepatorenal diseases in rats. BioMed Research International.
2015;
2015
:
563652
.
View Article PubMed Google Scholar -
P.
Gazzerro,
M. C.
Proto,
G.
Gangemi,
A. M.
Malfitano,
E.
Ciaglia,
S.
Pisanti,
M.
Bifulco.
Pharmacological actions of statins: A critical appraisal in the management of cancer. Pharmacological Reviews.
2012;
64(1)
:
102-146
.
View Article PubMed Google Scholar -
I. G.
Habeos,
P. G.
Ziros,
D.
Chartoumpekis,
A.
Psyrogiannis,
V.
Kyriazopoulou,
A. G.
Papavassiliou.
Simvastatin activates Keap1/Nrf2 signaling in rat liver. Journal of Molecular Medicine.
2008;
86(11)
:
1279-1285
.
View Article PubMed Google Scholar -
M.
Hu,
B. M.
Cheung,
B.
Tomlinson.
Safety of statins: An update. Therapeutic Advances in Drug Safety.
2012;
3(3)
:
133-144
.
View Article PubMed Google Scholar -
C.
Ip,
D. J.
Lisk.
Modulation of phase I and phase II xenobiotic-metabolizing enzymes by selenium-enriched garlic in rats. Nutrition and Cancer.
1997;
28(2)
:
184-188
.
View Article PubMed Google Scholar -
A.
Izzotti,
A.
Camoirano,
C.
Cartiglia,
C. J.
Grubbs,
R. A.
Lubet,
G. J.
Kelloff,
S.
De Flora.
Patterns of DNA adduct formation in liver and mammary epithelial cells of rats treated with 7,12-dimethylbenz(a)anthracene, and selective effects of chemopreventive agents. Cancer Research.
1999;
59(17)
:
4285-4290
.
PubMed Google Scholar -
S.
Kalantari,
M.
Naghipour.
Statin therapy and hepatotoxicity: Appraisal of the safety profile of atorvastatin in hyperlipidemic patients. Advanced Biomedical Research.
2014;
3(1)
:
168-178
.
View Article PubMed Google Scholar -
V. B.
Khyade.
Influence of Sibinin on DMBA Induced Hepatotoxicity and Free-Radical Damage in Norwegian Rat, Rattus norvegicus (L). International Journal of Current Microbiology and Applied Sciences.
2017;
6(1)
:
324-338
.
View Article Google Scholar -
R.
Kumar,
R.
Kaur,
A. P.
Singh,
S.
Arora.
Diminution of hepatic response to 7, 12-dimethylbenz(α)anthracene by ethyl acetate fraction of Acacia catechu willd through modulation of xenobiotic and anti-oxidative enzymes in rats. PLoS One.
2014;
9(2)
:
e90083
.
-
H.
Leaver,
T.
Keng Lim,
P.
Thomson,
J.
Leaver,
A. M.
Choy,
C. C.
Lang.
Compliance to recommended liver function monitoring in patients on statin therapy. Cardiovascular Therapeutics.
2009;
27(2)
:
96-100
.
View Article PubMed Google Scholar -
B.
Liu,
Z.
Yi,
X.
Guan,
Y. X.
Zeng,
F.
Ma.
The relationship between statins and breast cancer prognosis varies by statin type and exposure time: A meta-analysis. Breast Cancer Research and Treatment.
2017;
164(1)
:
1-11
.
View Article PubMed Google Scholar -
A. B.
Mulder,
H. J.
van Lijf,
M. A.
Bon,
F. A.
van den Bergh,
D. J.
Touw,
C.
Neef,
I.
Vermes.
Association of polymorphism in the cytochrome CYP2D6 and the efficacy and tolerability of simvastatin. Clinical Pharmacology and Therapeutics.
2001;
70(6)
:
546-551
.
View Article PubMed Google Scholar -
H. D.
Nelson,
M. E.
Smith,
J. C.
Griffin,
R.
Fu.
Use of medications to reduce risk for primary breast cancer: A systematic review for the U.S. Preventive Services Task Force. Annals of Internal Medicine.
2013;
158(8)
:
604-614
.
-
H.
Ohkawa,
N.
Ohishi,
K.
Yagi.
Assay for lipid peroxides in animal tissues by thiobarbituric acid reaction. Analytical Biochemistry.
1979;
95(2)
:
351-358
.
View Article PubMed Google Scholar -
H. J.
Pan,
H. T.
Chang,
C. H.
Lee.
Association between tamoxifen treatment and the development of different stages of nonalcoholic fatty liver disease among breast cancer patients. Journal of the Formosan Medical Association.
2016;
115(6)
:
411-417
.
View Article PubMed Google Scholar -
D.
Pradelli,
D.
Soranna,
L.
Scotti,
A.
Zambon,
A.
Catapano,
G.
Mancia,
G.
Corrao.
Statins and primary liver cancer: A meta-analysis of observational studies. European Journal of Cancer Prevention.
2013;
22(3)
:
229-234
.
View Article PubMed Google Scholar -
G. A.
Rao,
P. K.
Pandya.
Statin therapy improves sustained virologic response among diabetic patients with chronic hepatitis C. Gastroenterology.
2011;
140(1)
:
144-152
.
View Article PubMed Google Scholar -
S. P.
Robinson,
S. M.
Langan-Fahey,
V. C.
Jordan.
Implications of tamoxifen metabolism in the athymic mouse for the study of antitumor effects upon human breast cancer xenografts. European Journal of Cancer & Clinical Oncology.
1989;
25(12)
:
1769-1776
.
View Article PubMed Google Scholar -
C.
Stancu,
A.
Sima.
Statins: Mechanism of action and effects. Journal of Cellular and Molecular Medicine.
2001;
5(4)
:
378-387
.
View Article PubMed Google Scholar -
W.
Wang,
C.
Zhao,
J.
Zhou,
Z.
Zhen,
Y.
Wang,
C.
Shen.
Simvastatin ameliorates liver fibrosis via mediating nitric oxide synthase in rats with non-alcoholic steatohepatitis-related liver fibrosis. PLoS One.
2013;
8(10)
:
e76538
.
View Article PubMed Google Scholar
Comments
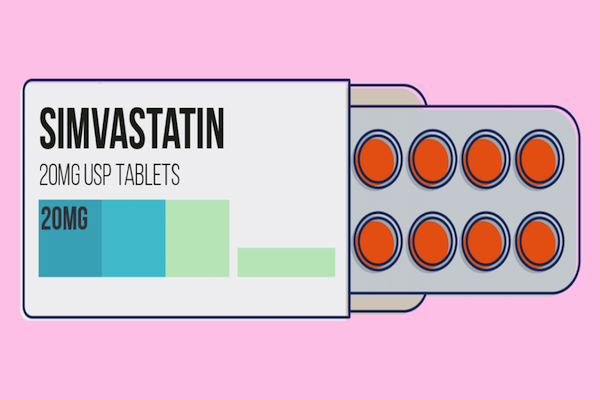
Downloads
Article Details
Volume & Issue : Vol 5 No 3 (2018)
Page No.: 2064-2077
Published on: 2018-03-13
Citations
Copyrights & License

This work is licensed under a Creative Commons Attribution 4.0 International License.
Search Panel
Pubmed
Google Scholar
Pubmed
Google Scholar
Pubmed
Google Scholar
Pubmed
Search for this article in:
Google Scholar
Researchgate
- HTML viewed - 7063 times
- Download PDF downloaded - 2325 times
- View Article downloaded - 0 times