Toxicological and melanin synthesis effects of Polygonum multiflorum root extracts on zebrafish embryos and human melanocytes
Abstract
Introduction
For a long time, Polygonum multiflorum (PM) has been traditionally used to treat various systemic diseases and biological conditions, including antioxidation Lv et al.,2007, radical scavenging activity Chen et al., 1999, lipid regulation Wang et al.,2012. In particular, it has been used to induce hair follicle growth as treatment for early gray hair Begum et al., 2015Park et al., 2011Sun et al., 2013. Nowadays, zebrafish is a popular model to study developmental and cellular biology Frank and Lisanti,2006Gousuddin et al., 2015. Zebrafish has been used as an ideal model for study of melanin formation and dispersion Parichy et al., 2000Rawls et al., 2001. So far, there have been limited studies focusing on the evaluation of toxicological and melanin synthesis effects of PM in vitro as well as in vivo. In this study, phytochemicals from PM roots were extracted into different solvents with gradually increasing polarity, including n-hexane, ethyl acetate and distilled water. The extracts were tested for their effects on teratogenic defects/deaths, on melanin formation in human melanocytes, and on pigmentation in zebrafish embryos/larvae.
Materials and methods
Ethics statement
For experiments in this study, we used zebrafish embryos and larvae at early periods of development, from 1 to 4 days after fertilization (no more than 5 days old). Therefore, no license was required by OECD guidelines (OECD, 1992 and 2013), or the Council of Europe (1986)- Directive 86/609/EEC (Louhimies, 2002), which regulates the use of animals in scientific experiments.
Preparation of root extracts
PM roots were obtained from Vietnam Pharmacy Institute. Fresh roots of PM were cleaned and washed thoroughly with water and re-washed with distilled water. The washed roots were dried in the shade, powdered mechanically, and sieved through a mesh. For preparation of organic solvent extracts, 5 g of powdered material was refluxed in 1/10 w/v in a soxhlet apparatus for 1 h. The resulting extract was filtered and pooled, and then the solvent was removed under reduced pressure at 40±5 °C using a rotary flash evaporator. The effectiveness of the extraction procedure is depicted in Table 1. Three types of organic solvents were used consecutively with gradually increasing polarity; these included nhexane, ethyl acetate (EtAc) and distilled water (DW).
Cell culture
Normal Human Primary Epidermal Melanocytes (code: ATCC® PCS-200-013™) were purchased from ATCC (Manassas, VA). Melanocytes were cultured in Dermal Cell Basal Medium (code: PCS-200-030™; ATCC), supplemented with Melanocyte Growth Kit (code: PCS-200-041™; ATCC) and 1% penicillin/streptomycin (code: ATCC®30-2300™, ATCC) at 37°C, 5% CO2.
Zebrafish culture and embryos selection
Adult zebrafish wild type strain AB (Danio rerio) (ZIRC, Eugene, OR) Lawson and Weinstein, 2002 was maintained in the zebrafish facility of the Animal Laboratory. The fish were cultured in glass rectangular pools measuring 40 cm (wide) x 50 cm (long) x 30 cm (high). Several pools of adult fish were bred individually for each assay. After sorting, embryos from pools with high fertility (≥80%) were mixed and used for subsequent experiments. Experiments were validated only when the survival rate of the controls was ≥90% at 4 days post fertilization (dpf). Fish were reared in a Tecniplast recirculating system under 14:10-h light/dark photocycle. The day before breeding, males and females were placed in breeding chambers with a separator to prevent undesired spawning. The next morning, fish were placed in fresh water system and the separator was removed to allow mating. Eggs were collected after 2 h and placed in E3 medium (5 mM NaCl, 0.17 mM KCl, 0.4 mM CaCl2, and 0.16 mM MgSO4) containing 0.01% methylene blue. The point of divider removal and mating start was marked as 0 hours post-fertilization (0 hpf); the breeding date was marked as 0 dpf. At around 3-4 hpf, eggs were screened and sorted under a stereoscope to remove the unfertilized and/or abnormal ones. Healthy embryos that showed normal cleavage were distributed into 6-well plates at 25 embryos/well for subsequent experiments.
Chemical exposure and embryo observation
Zebrafish embryos were divided into five groups, which included: (1) a negative control group for toxicity testing, (2) a group for investigating the toxicity of EtAc on development of embryos, (3) a group for investigating the toxicity/teratogenicity of PM-E on development of embryos, (4) a group for investigating the toxicity/teratogenicity of PM-W on development of embryos, and (5) a group for investigating the effect of PM-W on melanin formation. Fish Embryo Acute Toxicity (FET) was determined according to OECD test guidelines (OECD, 1992 and 2013) or equivalent guidelines. Briefly, after sorting of embryos, E3 medium was replaced with test solution and incubated at 28°C. Test solutions were renewed daily until 4 dpf. Embryos/larvae were observed under a stereoscope and all embryonic morphology and lethality were recorded. At 3 and 4 dpf, the embryos/larvae were photographed using an SZX10 stereomicroscope coupled with an XC50 camera (Olympus). Teratogenicity was assessed by determining the percentage of embryos/larvae with morphological defects over surviving ones. Phenotypes were compared with those described previously Kimmel et al., 1995.
Typical defects occurring in the zebrafish embryos when exposed to chemicals are: (1) edema, (2) hemovascular defects, (3) necrosis, (4) abnormal trunk, and (5) retardation. Edema refers to the abnormal accumulation of fluid inside the embryo, causing the skin around the affected organ to swell and internal structures to be pressed into abnormal shape. The most common types of edema are heart and yolk sac edema. Hemovascular defects consist of two main types: hemostasis and hemorrhage; both appear as red dots due to local red blood cell accumulation. Hemostasis is the accumulation of blood inside the cardiovascular system due to obstruction by a blood clot (thrombosis) or by blood vessel narrowing (stenosis). Hemorrhaging refers to bleeding which causes blood to accumulate outside of the cardiovascular system. The third defect (necrosis) refers to cell and tissue death caused by external factors such as toxins. Lastly, abnormal trunk refers to a curved/short tail/body and retardation refers to delayed developmental processes Lawson and Weinstein, 2002Pruvot et al., 2012Sylvain et al., 2010Tsay et al., 2007.
All experiments were repeated in triplicate (n = 25 embryos for each test). Data was calculated to determine indices, including median lethal concentration (LC50), median effective concentration (EC50), and the teratogenic index (TI; defined as the ratio between LC50 and EC50). If the TI value is lower than 1.0 (< 1.0), it means the tested substance is an agent which induces death of embryos/larvae; if the TI value is greater than 1.0 (> 1.0), it means the tested substance is a teratogenic agent for embryos/larvae.
Gene analysis
Total RNA of zebrafish embryos/larvae were isolated using a High Pure RNA Kit (Thermo Fisher Scientific, Waltham, MA), according to the manufacturer’s protocol. The cDNA was then synthesized by reverse transcription of total RNA using Super-scriptTMIII reverse transcriptase, according to a previously published protocol Dinh Thang et al., 2015Nguyen et al., 2015. Real-time quantitative RT-PCR with SYBR green was performed using power SYBR1 Green PCR master mix (Applied Biosystems, i.e. Thermo Fisher Scientific) in an ABI Prism 7500 sequence detection system (Applied Biosystems, i.e. Thermo Fisher Scientific). The transcript level tyrosinase was adjusted through the transcript expression level of housekeeping genes ef1α (for zebrafish) and GAPDH (for human melanocytes). Then, the PCR products were loaded on agarose gel for electrophoresis.
Sequences of primers for zebrafish were as follows:
Primers for tyrosinase, forward primer: 5’- CGCAGATGAACAATGGCTC-3’ and reverse primer: 5-AGCAGATACACCCGATGCC-3’
Primers for ef1α, forward primer: 5’- CTGGAGGCCAGCTCAAACAT-3’ and reverse primer: 5’- ATCAAGAAGAGTAGTACCGCTAGCATTAC-3’.
Sequences of primers for human were as follows:
Primers for tyrosinase, forward primer: 5’- TTGCCTGAGTTTGACCCAAT-3’ and reverse primer: 5’-GCATCCGCTATCCCAGTAAG-3’;
Primers for GAPDH, forward primer: 5’- CGGAGTCAACGGATTTGGTCGTAT-3’ and reverse primer: 5’-AGCCTTCTCCATGGTGGTGAAGAC-3’.
Total melanin content
The melanin content was determined according to previous publications Jiang et al.,2009Meyer et al., 2009. Briefly, zebrafish embryos/larvae were homogenized in 1 M NaOH and incubated at 80°C for 30 min followed by centrifugation at 1,500 rpm for 5 min. The supernatant was collected for absorbance measurement using dual wavelengths at 415 and 400 nm. Melanin has a maximum absorbance at 415 nm; however, blood cells can also strongly absorb light at 400 and 415 nm. Therefore, to minimize the interference of blood cells when determining melanin concentration, we first measured absorbance at 400 and 415 nm; the real absorbance of melanin = absorbance at 415 nm – absorbance at 400 nm. When measuring melanin concentration in melanocytes in vitro, the absorbance was measured directly at the single wavelength of 415 nm, as there was no effect from blood cells.
Statistical analysis
Statistical analysis was performed according to methods previously described Dinh Thang et al., 2015Nguyen et al., 2015. For toxicity/teratogenicity tests on zebrafish embryos, all statistical analyses, including regressions and comparison tests, were carried out using GraphPad Prism v.5.04 for Windows (GraphPad Software, La Jolla, CA). Percentages of dead/defective embryos were plotted against the logtransformed test concentrations of each substance. Sigmoidal concentration-response curves were obtained by fitting those data to the four-parameter equation:
Y = B o t t o m + ( Top − Bottom ) 1 + 10 ( logXC50 X ) − HillSlope
“Top” and “bottom”, respectively, represent the lowest and highest y-value (% dead/defective); “XC50” is either LC50 or EC50 concentration, and “HillSlope” refers to the steepness of the curve at the inflection point. LC50 and EC50 values for each substance were extracted from their corresponding equations. Results from 3 independent experiments in each group were statistically analyzed by Student’s ttest. The SPSS (version 18) software package (SPSS Japan Inc.) was used for statistical analyses, and the significance level was set at p < 0.05.
Results
Extraction efficiencies of PM roots in different solvents
Phytochemicals in the PM roots were extracted into 3 different solvents with increasing of polar powers: nhexane, EtAc and DW. The extraction efficiencies are depicted in Table 1 . In general, the results showed that the percentage of phytochemicals dissolved in DW was dominant (29.6 %) compared to that in n-hexane (1.28 %) and ethyl acetate (0.98 %) ( Table 1 ).
Toxicity of ethyl acetate (EtAc) on development of zebrafish embryos
Firstly, we performed experiments to evaluate the safe doses of EtAc for development of zebrafish embryos. EtAc at various concentrations of 0, 0.05, 0.075, 0.10, 0.125, 0.15, 0.20, and 0.25 % (w/v) in E3 medium were used. The summary of observed malformations of zebrafish embryos/larvae with different exposure times to EtAc are presented in Table 2 .
At concentrations below 0.1 %, EtAc had no effect on the development of zebrafish embryos/larvae. However, at 0.1 % concentration, EtAc started to cause abnormal development with slight yolk sac edema and haemovascular defects without any death of embryos/larvae. At a concentration of 0.25 %, EtAc caused death of all embryos embryos at 4 dpf. Moreover, at concentrations of 0.125, 0.15 and 0.20 %, EtAc strongly induced toxicological effects on embryos/larvae, with a dose-dependent effect on embryo development (the higher the dose, the greater the defects). After 24 hpf, malformation of embryos/larvae started to appear, including development retardation, heart oedema, yolk sac edema. Additionally, hemovascular defects were seen with appearance of red dot accumulations and yolk/head/body necrosis ( Figure 1A,D,G ); meanwhile the embryos/larvae in E3 medium developed normally without any defects (data not shown). After 3 dpf ( Figure 1E,B,H ) or 4 dpf ( Figure 1C,F,K ), many types of malformations occurred in the embryos/larvae and the number of embryos/larvae death rose with time exposure and increasing concentrations of EtAc. Statistical analysis of the data by GraphPad software yielded concentration-response curves for lethality and developmental defects ( Figure 1L ). LC50 and EC50 values at 4 dpf, calculated based on the respective curve equations, were 0.141 % (w/v) and 0.138 % (w/v), respectively. The teratogenic index (TI) was 1.02. The results suggest that EtAc at concentrations over 0.10 % are teratogenic for zebrafish larvae at 4 dpf.
Toxicity of PM root extracts in ethyl acetate (PM-E) and effect on development of zebrafish embryos Next, we investigated the effect of PM-E (0.075 % ethyl acetate) on development of zebrafish embryos/larvae. The toxic effects of PM-E at various concentrations (0, 10, 20, 30, 40, 50, 80, and 100 mg/L) on embryos/larvae were investigated. Embryos/larvae were exposed to PM-E solution for 4 dpf. The observed malformations on embryos/larvae are summarized in Table 3 .
At concentrations below 40 mg/L, PM-E did not affect the development of embryos/larvae and there were no induced morphological defects. At the concentration of 40 mg/L, some morphological defects occurred; however, there was no death of embryos/larvae ( Figure 2A ). At the concentrations ranging from 50 to 100 mg/L, both morphological defects and deaths occured in embryos/larvae in a dose-dependent and timedependent manner ( Figure 2B-H ). Statistical analysis of the data yielded concentration-response curves for lethality and developmental defects ( Figure 2K ). LC50 and EC50 values at 4 dpf, calculated based on the respective curve equations, were 70.94 mg/L and 49.75 mg/L, respectively; the TI was 1.43. The results suggest that PM-E at concentrations over 40 mg/L are teratogenic for zebrafish larvae/embryos at 4 dpf.
Toxicity of PM root extracts in distilled water (PMW) on development of zebrafish embryos
We then examined the toxicity of PM-W at various concentrations (0, 70, 87.5, 105, 122.5, 140, 157.5, and 175 mg/L) on development of zebrafish embryos/larvae. Embryos/larvae were exposed to PMW for 4 pdf. The observed malformations on zebrafish embryos/larvae are summarized in Table 4 .
At the concentrations of 0% (negative control), 70 mg/L and 87.5 mg/L, embryos/larvae developed in a normal manner without any morphological defects (data not shown). At the concentration of 105 mg/L, some morphological defects started to occur; however, there was no death of embryos/larvae ( Figure 3 ). At the concentrations ranging from 122.5 to 175 mg/L, both morphological defects and death of embryos/larvae occurred ( Figure 3B-E ).
The results also revealed that at 1 dpf, no morphological defect was observerd; at 2 dpf, only one morphological defect (yolk sac edema) was observed (at high concentrations of 157.5 and 175 mg/L but not at low concentrations, i.e. below 157.5 mg/L). However, at 4 dpf, many morphological defects were observed for all tested concentrations ranging from 105 to 175 mg/L. Statistical analysis of the data yielded concentration-response curves for lethality and developmental defects ( Figure 3F ). LC50 and EC50 values at 4 dpf, calculated based on the respective curve equations, were 135.7 mg/L and 225.1 mg/L, respectively; the TI was 0.63. These results indicate that PM-W, at concentrations over 105 mg/L, is an agent which causes death of zebrafish embryos/larvae at 4 dpf.
PM-W induced melanin formation in zebrafish embryos/larvae
Based on the above toxicity tests, PM-W (at the safe doses) was evaluated for its effect on pigmentation of zebrafish embryos/larvae. The results (from observations under the microscope) showed that treatment with PM-W, at a concentration of 87.5 mg/L, significantly promoted pigment development of zebrafish embryos/larvae at 4 dpf ( Figure 4B ), as compared to that for control embryos/larvae ( Figure 4A ). The amount of melanin formed in the zebrafish embryos/larvae at 4 dpf were measured and demonstrated in Figure 4C; the embryos/larvae exposed to 87.5 mg/L PM-W had a 2.2-fold greater amount of melanin, compared to that of the control embryos/larvae ( Figure 4C ).
PM-W promoted pigmentation of zebrafish embryos/larvae via activation of tyrosinase
We then investigated the effect of PM-W on transcript levels of tyrosinase (which plays a critical role in synthesis of melanin) and ef1α (an internal control). Zebrafish embryos/larvae were exposed to E3 medium as a negative control (lane 1), and to PM-W at various concentrations (25 mg/L, 70 mg/L and 87.5 mg/L) for 4 dpf. After 4 dpf, embryos/larvae were collected for RNA isolation, cDNA synthesis and gene analysis. Our results indicated that 25 mg/L PM-W did not affect the transcription level of tyrosinase (lane 3, Figure 5A,B ). However, at the concentrations of 70 mg/L (lane 4, Figure 5A,B ) and 87.5 mg/L (lane 5, Figure 5A,B ), PM-W significantly induced the transcription level of tyrosinase in embryos/larvae, up to 1.83- and 1.75- fold compared to the negative control (lane 2, Figure 5A,B ). Meanwhile, PM-W at the tested concentrations did not change the transcription level of ef1α ( Figure 5C,D ).
PM-W increased melanin synthesis of melanocytes via activation of tyrosinase
Next, we investigated the effect of PM-W on the biosynthesis of melanin in human melanocytes. The tested concentrations of PM-W were 0 (negative control), 50, 100, 250, 500, and 1000 μg/mL. First, we examined the cytotoxicity of PM-W on melanocytes. The results showed that PM-W at high concentrations of 250, 500 and 1000 μg/mL (but not at low concentrations of 50 and 100 μg/mL) induced cytotoxicity in 36 %, 47 % and 52 % of the melanocytes, respectively ( Figure 6A,B ). The ability of PM-W to stimulate melanin synthesis in melanocytes was examined next ( Figure 6C,D ). The data showed that PM-W at 100 μg/mL (the safe dose) significantly induced total melanin formation in melanocytes up to 179 %. Total melanin was measured and presented as a picture ( Figure 6C ) and graph ( Figure 6D ).
Moreover, we examined the effect of PM-W on the transcript levels of tyrosinase and GAPDH (another internal control). Our results revealed that PM-W at concentrations of 50 and 100 μg/mL increased transcript expression of tyrosinase up to 1.63- and 1.71-fold, respectively ( Figure 6F-G ). Our results show that PM-W has a low toxicity toward melanocytes but that it can promote melanin synthesis via activation of tyrosinase.
Discussion
The use of plant extracts as drugs is increasing in Asia. However, there are concerns that hazardous substances exist in these extracts, which may have serious effects but have been understudied. Therefore, it is necessary to have toxicity testing before use. The zebrafish embryo is an emerging and useful model system for toxicity testing in the laboratory. Its advantages are rapid development (at the rate of about 50-300 embryos/pair/week), high availability and easy observation of transparent embryos (Kimmel et al., 1995). More importantly, because the developmental process of a zebrafish embryo represents that of other vertebrates with highly similar embryogenesis and organogenesis, it is considered a complex and independent organism with a “nonanimal” status. In addition, the well-characterized zebrafish genome shows many similarities with higher vertebrates, including humans Howe et al., 2013, especially with regard to structure and function of genes, such as those of the CYP family involved in drug metabolism in mammals Rubinstein, 2006. Therefore, zebrafish embryos are an ideal vertebrate model for melanin formation and dispersion tests, as well as for toxicity testing without raising any ethical issues Lessman, 2011Lieschke and Currie,2007OECD.
PM root extracts have been used in folk medicine for treatment of various diseases, such as those related to pigmentation disorders including early graying of hair. Recently, there have been several studies showing that PM has potential effects on melanin synthesis in vitro (e.g. in B16 cells) Hu, 2008, as well as in vivo (e.g. mouse models) Sun, et al., 2013. However, so far the toxicity of PM root extracts have not been well elucidated. In our study, we extracted phytochemicals in the roots of PM using different solvents, including n-hexane, ethyl acetate and distilled water, and examined the toxicological and melanin biosynthesis effects of these extracts on development of zebrafish larvae/embryos. Our results showed that all these extracts have toxic properties and cause abnormal development as well as teratogenic defects of zebrafish larvae/embryos. Among the two extracts, PM-W had a lower toxicity effect than PM-E on the embryos/larvae. Importantly, our results showed that PM-W induced melanin formation in embryos/larvae and human melanocytes via activation of tyrosinase.
There are many signaling pathways which contribute to the regulation of biosynthesis of melanin in melanocytes; these include MC1R/cAMP/MITF/Tyrosinase, β-catenin/MITF/Tyrosinase, and RTK/ERK/MITF/Tyrosinase Amsen, 2009Bae-Harboe and Park, 2012Schiaffino, 2010. Among these signaling pathways, tyrosinase is the most important since it catalyzes the metabolism of DOPA into melanin as the final product Gray-Schopfer et al., 2007Millington, 2013Peters et al., 2011Slominski,2004. In this study, we examined the expression level of tyrosinase to understand the effects of PM root extracts on melanin formation in the zebrafish embryos/larvae model and melanocyte cell model. Our data revealed that PM-W enhances the transcript expression level of tyrosinase, not only in zebrafish embryos/larvae but also in human melanocytes. Our results lend support to the traditional use of PM-W for treating early gray hair development in humans.
Conclusion
In summary, results from our study indicate that PM root extracts (especially in water) is a very potent agent for treatment of diseases related to loss of pigmentation. Due to the toxicological and biological effects of PM-W on embryos of zebrafish, however, more studies are warranted before its use as folk medicine for humans, especially use in pregnant women, to avoid possible negative effects of PM root extracts on the fetus.
References
-
E.M.
Amsen.
Studies of Proteins that Regulate Melanin Synthesis and Distribution (University of Toronto). 2009
.
-
Y.-S.C.
Bae-Harboe,
H.-Y.
Park.
Tyrosinase: A Regulatory Protein for Cutaneous Pigmentation. Journal of Investigative Dermatology.
2012;
132
:
2678-2680
.
-
S.
Begum,
L.-J.
Gu,
M.-R.
Lee,
Z.
Li,
J.-J.
Li,
M.J.
Hossain,
Y.-B.
Wang,
C.K.
Sung.
In vivo hair growthstimulating effect of medicinal plant extract on BALB/c nude mice. Pharmaceutical Biology.
2015;
53
:
1098-1103
.
-
Y.
Chen,
M.
Wang,
R.T.
Rosen,
C.-T.
Ho.
2,2- Diphenyl-1-picrylhydrazyl Radical-Scavenging Active Components from Polygonum multiflorum Thunb. J Agric Food Chem.
1999;
47
:
2226-2228
.
-
N.
Dinh Thang,
I.
Yajima,
M.Y.
Kumasaka,
M.
Iida,
T.
Suzuki,
M.
Kato.
Deltex-3-like (DTX3L) stimulates metastasis of melanoma through FAK/PI3K/AKT but not MEK/ERK pathway. Oncotarget.
2015;
6
:
14290-14299
.
-
P.G.
Frank,
M.P.
Lisanti.
Zebrafish as a Novel Model System to Study the Function of Caveolae and Caveolin-1 in Organismal Biology. The American Journal of Pathology.
2006;
169
:
1910-1912
.
-
M.
Gousuddin,
S.
Roohi,
V.L.
Pattankar.
Common lesions of uterus and cervix with mast cell profile. 2015
.
-
V.
Gray-Schopfer,
C.
Wellbrock,
R.
Marais.
Melanoma biology and new targeted therapy. Nature.
2007;
445
:
851-857
.
-
K.
Howe,
M.D.
Clark,
C.F.
Torroja,
J.
Torrance,
C.
Berthelot,
M.
Muffato,
J.E.
Collins,
S.
Humphray,
K.
McLaren,
L.
Matthews.
The zebrafish reference genome sequence and its relationship to the human genome. Nature.
2013;
496
:
498-503
.
-
D.-N.
Hu.
Methodology for Evaluation of Melanin Content and Production of Pigment Cells in Vitro. Photochemistry and Photobiology.
2008;
84
:
645-649
.
-
Z.
Jiang,
J.
Xu,
M.
Long,
Z.
Tu,
G.
Yang,
G.
He.
2, 3, 5, 4′-tetrahydroxystilbene-2-O-β-d-glucoside (THSG) induces melanogenesis in B16 cells by MAP kinase activation and tyrosinase upregulation. Life Sciences.
2009;
85
:
345-350
.
-
C.B.
Kimmel,
W.W.
Ballard,
S.R.
Kimmel,
B.
Ullmann,
T.F.
Schilling.
Stages of embryonic development of the zebrafish. Dev Dyn.
1995;
203
:
253-310
.
-
N.D.
Lawson,
B.M.
Weinstein.
In Vivo Imaging of Embryonic Vascular Development Using Transgenic Zebrafish. Developmental Biology.
2002;
248
:
307-318
.
-
C.A.
Lessman.
The developing zebrafish (Danio rerio): A vertebrate model for high-throughput screening of chemical libraries. Birth Defects Research Part C: Embryo Today: Reviews.
2011;
93
:
268-280
.
-
G.J.
Lieschke,
P.D.
Currie.
Animal models of human disease: zebrafish swim into view. Nat Rev Genet.
2007;
8
:
353-367
.
-
L.
Lv,
X.
Gu,
J.
Tang,
C.
Ho.
Antioxidant activity of stilbene glycoside from Polygonum multiflorum Thunb in vivo. Food Chemistry.
2007;
104
:
1678-1681
.
-
K.C.
Meyer,
T.
Brzoska,
C.
Abels,
R.
Paus.
The α- melanocyte stimulating hormone-related tripeptide K(D)PT stimulates human hair follicle pigmentation in situ under proinflammatory conditions. British Journal of Dermatology.
2009;
160
:
433-437
.
-
G.W.M.
Millington.
Mutations of the BRAF gene in human cancer, by Davies et al. (Nature 2002; 417: 949-54). Clinical and Experimental Dermatology 38, 222-223.
2013
.
-
D.T.
Nguyen,
T.N.
Phan,
M.Y.
Kumasaka,
I.
Yajima,
M.
Kato.
. Asian Pacific Journal of Cancer Prevention.
2015;
16
:
699-705
.
-
null.
OECD Test No. 423: Acute Oral toxicity - Acute Toxic Class Method. OECD Publishing.
.
-
D.M.
Parichy,
E.M.
Mellgren,
J.F.
Rawls,
S.S.
Lopes,
R.N.
Kelsh,
S.L.
Johnson.
Mutational Analysis of Endothelin Receptor b1 (rose) during Neural Crest and Pigment Pattern Development in the Zebrafish Danio rerio. Developmental Biology.
2000;
227
:
294-306
.
-
H.-J.
Park,
N.
Zhang,
D.K.
Park.
Topical application of Polygonum multiflorum extract induces hair growth of resting hair follicles through upregulating Shh and β-catenin expression in C57BL/6 mice. Journal of Ethnopharmacology.
2011;
135
:
369-375
.
-
E.M.
Peters,
D.
Imfeld,
R.
Gräub.
Graying of the human hair follicle. Journal of cosmetic science.
2011;
62
:
121
.
-
B.
Pruvot,
Y.
Quiroz,
A.
Voncken,
N.
Jeanray,
A.
Piot,
J.A.
Martial,
M.
Muller.
A panel of biological tests reveals developmental effects of pharmaceutical pollutants on late stage zebrafish embryos. Reproductive Toxicology.
2012;
34
:
568-583
.
-
J.F.
Rawls,
E.M.
Mellgren,
S.L.
Johnson.
How the Zebrafish Gets Its Stripes. evelopmental Biology.
2001;
240
:
301-314
.
-
A.L.
Rubinstein.
Zebrafish assays for drug toxicity screening. Expert Opinion on Drug Metabolism & Toxicology.
2006;
2
:
231-240
.
-
M.V.
Schiaffino.
Signaling pathways in melanosome biogenesis and pathology. he International Journal of Biochemistry & Cell Biology.
2010;
42
:
1094-1104
.
-
A.
Slominski.
Melanin Pigmentation in Mammalian Skin and Its Hormonal Regulation. Physiological Reviews.
2004;
84
:
1155-1228
.
-
Y.N.
Sun,
L.
Cui,
W.
Li,
X.T.
Yan,
S.Y.
Yang,
J.I.
Kang,
H.K.
Kang,
Y.H.
Kim.
Promotion effect of constituents from the root of Polygonum multiflorum on hair growth. Bioorganic & Medicinal Chemistry Letters.
2013;
23
:
4801-4805
.
-
N.J.
Sylvain,
D.L.
Brewster,
D.W.
Ali.
Zebrafish embryos exposed to alcohol undergo abnormal development of motor neurons and muscle fibers. Neurotoxicology and Teratology.
2010;
32
:
472-480
.
-
H.-J.
Tsay,
Y.-H.
Wang,
W.-L.
Chen,
M.-Y.
Huang,
Y.-H.
Chen.
Treatment with sodium benzoate leads to malformation of zebrafish larvae. Neurotoxicology and Teratology.
2007;
29
:
562-569
.
-
M.
Wang,
R.
Zhao,
W.
Wang,
X.
Mao,
J.
Yu.
Lipid regulation effects of Polygoni Multiflori Radix, its processed products and its major substances on steatosis human liver cell line L02. Journal of Ethnopharmacology.
2012;
139
:
287-293
.
Comments
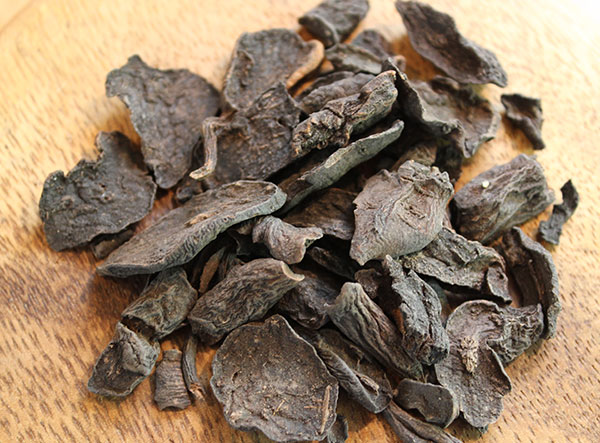
Downloads
Article Details
Volume & Issue : Vol 3 No 09 (2016)
Page No.: 808-818
Published on: 2016-09-29
Citations
Copyrights & License

This work is licensed under a Creative Commons Attribution 4.0 International License.
Search Panel
Pubmed
Google Scholar
Pubmed
Google Scholar
Pubmed
Search for this article in:
Google Scholar
Researchgate
- HTML viewed - 7938 times
- Download PDF downloaded - 1702 times
- View Article downloaded - 17 times