Abstract
Background: The present study compares the efficacy of quetiapine fumarate (QF) and QF-loaded solid lipid nanoparticles (QFSLN) as antipsychotic drugs for schizophrenia.
Methods: To induce schizophrenia-like symptoms, a group of rats was injected intraperitoneally (i.p.) with ketamine (25mg/kg b.w.) for 1 week to establish a rat model of schizophrenia. The incidence of schizophrenic symptoms was estimated to be equivalent to the control group. To estimate the pronounced antipsychotic effect of QF, a low dose (LD) of 10 mg/kg b.w. and a high dose (HD) of 30 mg /kg b.w. were orally administrated to two groups of rats (designated L.QF and H.QF) for 3 weeks (2 weeks without ketamine injection; the last week with ketamine). To achieve the optimal therapeutic response of QF drug, 2 other groups of rats were administered orally the equivalent low and high doses of QF in its solid lipid nanoparticle form (L.QFSLN) and (H.QFSLN) for 3 weeks in the same manner. The treatments were given after 1 h of ketamine injection. To assess the effect of different doses of treatment on hyperlocomotion and cognitive impairment induced by ketamine, an open field test and passive avoidance test were conducted. In addition, excitatory and inhibitory amino acids, as well as catecholamines, were estimated in brain regions (cortex and hippocampus). The study was extended to estimate the side effects of different treatments on hepatorenal functions and lipid profile. Additionally, samples were subjected to immunohistochemical analysis.
Results: QFSLN treatment showed enhanced effect over QF in a dose-dependent manner with minimal side effects in schizophrenic rats. In addition, immunohistochemical examinations of brain tissues confirmed the biochemical data.
Introduction
Schizophrenia is a complex neuropsychiatric disease that consists of positive symptoms (delusions and hallucinations), negative symptoms (blunted affect and social withdrawal), and cognitive impairments Pickard, 2015. Previous investigations have shown an imbalance of several neurotransmitter systems in the pathophysiology of schizophrenia Arion et al., 2007De Oliveira et al., 2011. The dopaminergic pathway was the first to be studied in schizophrenia. Dopaminergic hyper-function manifests in the aforementioned positive symptoms. In addition to dopamine (DA), glutamate is one of the main neurotransmitters involved in schizophrenia pathophysiology De Oliveira et al., 2011. The scientific evidence of the involvement of glutamate is from the fact that phencyclidine (PCP) and ketamine are two N-methyl-D-aspartate (NMDA) glutamatergic receptor antagonist drugs which cause severe symptoms similar to those observed in schizophrenia Arion et al., 2007De Oliveira et al., 2011.
Ketamine has been widely used to induce characteristics of cognitive impairment seen in schizophrenia (e.g. difficulty in functions related to attention, memory and behavior). Indeed, ketamine induces schizophrenic-like psychotic symptoms in normal adult subjects Krystal et al., 1994. Moreover, Malhotra et al., (1996) estimated that sub-anesthetic doses of NMDA receptor antagonists (e.g. ketamine) induces a spectrum of behavioral responses in healthy human volunteers that resemble positive, negative and cognitive schizophrenic-like symptoms Malhotra et al., 1996.
Antipsychotic drugs are classified as typical or atypical antipsychotics. Typical antipsychotic such as chlorpromazine and haloperidol ameliorate only the positive signs. Atypical antipsychotics such as clozapine, quetiapine and risperidone are useful in treating the positive, negative and cognitive signs. Both typical and atypical antipsychotics can block DA receptors Bellino et al., 2006.
The blockade of DA receptor D2 in the mesolimbic area is considered to be responsible for the reverse of positive symptoms by antipsychotics. Atypical antipsychotics can also bind to serotonin (5-HT) receptors. QF is an atypical psychotropic agent that belongs to the thienobenzodiazepine class of schizophrenia drugs Barch et al., 2001. In 1985 scientists at AstraZeneca Pharmaceuticals developed QF, which was later approved by the US FDA in 1997 Riedel et al., 2007. Since then, QF has been used in the treatment of several mental disorders in more than 70 countries, such as Canada, Europe and Japan. Nevertheless, QF has poor oral bioavailability (about 9%) with a plasma half-life of 6 hours Mehnert and Mäder, 2001. Narala and Veerabrahma (2013) reported that the poor bioavailability of QF was solved by using the SLN formulation of QF Narala and Veerabrahma, 2013. The SLN form was optimized based on nanoparticles and drug release features Kreuter, 2001MuÈller et al., 2000.
Bcl-2, one of the key proteins that suppress cell apoptosis, was examined to discern the neuroprotective effects of the antipsychotic drugs. Up-regulation of Bcl-2 expression is a critical mechanism for cell survival Adams and Cory, 2001.
The aim of this study was to evaluate and compare QF and QFSLN as antipsychotic drugs to treat ketamine-induced schizophrenia-like symptoms in albino rats.
Materials - Methods
Materials
Drugs
Quetiapine and ketamine were purchased from the local Egyptian market. Ketamine was dissolved in normal saline and injected intraperitoneally (i.p.) at a dose of 25 mg/kg b.w. Malhotra et al., 1997. QF was orally administered to rats in two doses, 10 mg/kg b.w. (equivalent to therapeutic human daily dose) and 30mg/kg b.w., for 3 weeks. All other chemicals were of high-performance liquid chromatograph (HPLC) analytical grade and commercially available. Chemical preparation of QF loaded solid lipid nanoparticles (QFSLN): QFSLN were prepared by hot homogenization followed by ultrasonication. QF, solid lipid and lecithin were then dissolved in 10 ml of a mixture of methanol and chloroform (1:1). Organic solvents were completely removed using a rotary evaporator. The embedded lipid layer was melted by heating to 5ºC above the melting point of the lipid. An aqueous phase was prepared by adding the stabilizer Tween 80 in distilled water (1.5% w/v) and heating to the same temperature of the oil phase. The hot aqueous phase was added to the oil phase and homogenization was performed at 12,000 rpm using a homogenizer (DIAX 900 Heidolph, Germany) for 5min. The coarse oil in water emulsion was obtained by sonication using a probe (12T) sonicator (Vibracell Sonics, USA) for 20 min. QF-loaded SLN were finally obtained by allowing the hot nano-emulsion to cool at room temperature Blasi et al., 2013.
Animals
Thirty-six male albino rats weighing about 170 g (±10 g) were used in this study. Animals were obtained from the animal house of NODCAR, Egypt. They were kept under strict hygienic conditions and allowed free access to diet and tap water. They were acclimated to the environment for two weeks prior to the start of the experiment. The experimental animal protocol was approved by the ethical committee of NODCAR overseeing animal care and usage.
Experimental design
Rats were randomly divided into six groups (six rats each). Group 1 was the control (C) group wherein rats were fed a basal diet and injected i.p. with 0.2 ml of saline per rat; Group 2 was the ketamine (Ket) group wherein rats were injected i.p. with ketamine (25 mg/kg b.w.) daily for 1 week; Group 3 was the Low Dose QF (L.QF) treated group wherein rats were orally administered with QF (10 mg/kg b.w.) for 3 weeks; and Group 4 was the high dose QF (H.QF) treated group wherein rats were orally administered QF (30 mg/kg b.w.) for 3 weeks (2 weeks without ketamine and the last week with ketamine). Additionally, Group 5 was the low dose QF-loaded solid lipid nanoparticle (L.QFSLN) treated group wherein rats were orally administered with a daily dose of QFSLN (10 mg/ kg b.w.) for 3 weeks, and Group 6 was the high dose QF-loaded solid lipid nanoparticle (H.QFSLN) treated group wherein rats were orally administered with a daily dose of QFSLN (30 mg/kg. b.w.) for 3 weeks. At the third week, ketamine was injected i.p. (25 mg/kg b.w.) in groups 2-6.
At the end of the experiment, blood samples were collected from the retro-orbital plexus veins, rats were sacrificed, and serum samples were separated at 3000 rpm for collection and storage at -20ºC until analysis. Brain tissues were quickly removed from each animal and washed in ice-cold saline. Two brain tissue samples from each cortex and hippocampus area of each rat were used. One part was immersed in formalin for immunohistochemical evaluation and the other part was homogenized separately in 70% iced methanol to yield a 10% homogenate (w/v) solution, which was stored at -20ºC until further analysis.
Methods
Animal behavior
Open field test: To estimate locomotor activity, rats were placed in an open field installation El-Sisi, 2015, i.e. a square wooden field measuring 90x90x25 cm.
The wood of the equipment was covered with a plastic laminate (Formica, Cincinnati, OH), which prevents absorption of fluids (e.g. urine of rats). The platform was divided by black lines into 36 small squares (15x15 cm). The open field maze was cleaned between each rat test using 70% ethyl alcohol to bypass odor cues. The rats were transferred to the test room in their home cages and examined one at a time for 5 min each. Rats were handled by the base of their tails at all times. The rats were brought from their home cages and placed randomly into one of the four corners of the open field facing the center. The behavioral scores were estimated, in this analysis, by the total number of line crossings.
Passive avoidance test: Passive avoidance test was used to assess the impact of the different treatments on memory. The apparatus was comprised of identical illuminated and non-illuminated boxes. The illuminated compartment (20x20x20 cm) connected with the non-illuminated compartment (20x20x20 cm) by a guillotine door (5x5 cm). The floor of the non-illuminated compartment was composed of 2 mm stainless steel rods spaced 1cm apart. Each rat were gently placed into the illuminated compartment for an acquisition trial and the door between the two compartments was opened after 10 sec. The rat was placed again in the illuminated compartment for a retention trial. The time taken for a rat to enter the dark part after opening of the door was recorded as the latency time for both acquisition and retention trials. Latency for entering the dark compartment was recorded up to 150 sec. If a rat did not enter the dark chamber within 30 sec, the rat was removed and assigned a latency score of 150 sec. QF was given 1 h after the acquisition trial McLamb et al., 1990.
Biochemical analysis
Assessment of brain areas (cortex& hippocampus) amino acids: In the brain tissues, excitatory (glutamate) and inhibitory (gamma-Amniobutyric acid; GABA) amino acids were determined using HPLC methods Heinrikson and Meredith, 1984. Catecholamines norepinephrine (NE), serotonin (5-HT) and DA were estimated by HPLC methods according to Pagel et al., (2000) Pagel et al., 2000.
Assessment of liver function: The activities of serum alanine aminotransferase (ALT), aspartate aminotransferase (AST), gamma-glutamyl transferase (GGT), and creatine kinase (CK) were measured by commercial kits according to the method of IFCC (2010) Schumann et al., 2010. Alkaline phosphatase (ALP) was measured by a commercial kit according to the method of Tietz (1994) Tietz, 1994. Albumin concentration was measured by a commercial kit according to the method of Tietz (1995) Tietz, 1995.
Evaluation of kidney function: Determination of serum urea and creatinine were carried out by commercial kits according to the methods of Tietz (2005) and Levey et al., (2007), respectively Levey et al., 2007Tietz, 2005.
Determination of lipid profile: Serum total cholesterol, high-density lipoprotein (HDL) cholesterol, low-density lipoprotein (LDL) cholesterol, and triglycerides were measured by commercial kits following the methods described by NCEP (2001) Panel, 2001.
Immunohistochemistry (IHC)
Immunohistochemistry (IHC) for Bcl2 was performed. Sections were fixed in formalin and then embedded in paraffin. The sections were pre-treated using pressure cooker heat mediated antigen retrieval with sodium citrate buffer (pH 6) and then incubated at 1/1000 dilution for 15 min at room temperature. A goat anti-chicken biotinylated secondary antibody was used to detect the primary antibody and visualized using an HRP conjugated ABC system. The sections were counterstained with hematoxylin and mounted with DPX mounting medium.
Statistical analysis
Data values were expressed as mean ± S.E of 6 rats. One-way ANOVA tests (from SPSS version 23) were used to study the relationship between the different variables. P<0.05 was considered statistically significant Armitage, 2008.
Results
The QF-loaded nanoparticles were generated are shown in Figure 1 . The surface morphology was analyzed using scanning electronic microscope, which confirmed that nanoparticles were mostly spherical in shape ( Figure 1A , Figure 1B ). The nanoparticles had an approximate particle size of 134-162 nm ( Figure 1C ).
Animal behavior
The schizophrenic symptoms of rats were demonstrated using open field test ( Figure 2A ) and passive avoidance test ( Figure 2B ). The number of squares in the open field test were significantly increased (p<0.05) in the group of rats treated with ketamine; in addition the time spent in the dark room (latency time) in the passive avoidance test was significantly increased (p<0.05) with respect to the control group. These effects were more pronounced (p<0.05) in rats treated with QFSLN compared to QF. The data revealed that the observed effects were dose-dependent since the greatest effect was observed in the higher dose of treatments.
Biochemical analysis
The effects of the different treatments on DA, NE and 5-HT in brain hippocampus and cortex are represented in Table 1 . It is clear that administration of ketamine caused a significant (p<0.05) elevation of DP in both regions. NE was significantly (p<0.05) reduced in the cortex but unchanged in the hippocampus region, 5-HT was slightly (p>0.05) reduced in the hippocampus and significantly (p<0.05) increased in the cortex region, compared to the control group. On the other hand, QF administration ameliorated the disturbance of the neurotransmitters either at low or high doses while the effect was even more noticeable in rats treated with QFSLN, in a dose-dependent manner.
Table 2 illustrates the effect of different treatments on the level of GABA and glutamate in the cortex and hippocampus of brain tissues. From the table, it is clear that ketamine caused a reduction in the level of GABA in the cortex and hippocampus regions. Glutamate was reduced in the cortex and significantly (p<0.05) increased in the hippocampus. The different treatments ameliorated these values.
Figure 3 illustrates the effect of the various treatments on hepatorenal function, serum proteins and serum lipids after 3 weeks of treatment. In general, there was a slight (p>0.05) increase on the different parameters after treatment with H.QF and QFSLN (low & high doses); however, only treatment with ketamine induced a significant (p<0.05) increase in the level of serum CK.
Immunohistochemical (IHC) study
IHC analysis of Bcl2 in both the cortex and hippocampus of rats treated with QF showed a moderate positive expression of Bcl2, compared to the ketamine group that had negative expression in the cortex ( Figure 4 ). On the other hand, this effect was increased in rats treated with QFSLN, which showed strong positive expression in the hippocampus ( Figure 5 ). Moreover, the observed expression was dose-dependent, i.e. expression was strongest at the highest dose (30 mg/kg b.w.).
Discussion
Schizophrenia is a chronic and severe disorder of the brain that results from disturbance in the levels of neurotransmitters Wang et al., 2012.
Psychotomimetic effects of NMDA receptor antagonists in humans suggest that reduced NMDA receptor function may contribute to the pathophysiology of schizophrenia. The antagonists of the NMDA receptor used in this study was ketamine, known to induce a spectrum of behavioral effects that mimic positive, negative and cognitive symptoms of schizophrenia. The occurrence of schizophrenia was confirmed using passive avoidance test and open field test. Ketamine administration caused a significant increase in the locomotor activity of rats as shown in the open field test. Ketamine also caused depletion of memory as indicated by the increased time spent in the light box in the passive avoidance test (representing signs of positive symptoms and cognitive impairment). The data also showed a significant increase of CK enzyme activity after ketamine injection. CK is found mainly in brain, skeletal muscles and heart. Therefore, the increase of CK after 3 weeks of ketamine injection may be a result of the effect of ketamine on brain tissue and induction of schizophrenia. Furthermore, results from the study showed that administration of ketamine increased the levels of DA, NE, and 5-HT in areas of the brain. A reduction in the level of excitatory amino acids (e.g. glutamate) and inhibitory amino acids (e.g. GABA) were observed. Changes in dopaminergic activity are likely to be the primary cause of schizophrenia or positive symptoms of schizophrenia.
With respect to animal models, while the underlying neuropharmacological mechanisms may be the same in humans and rodents, changes in DA-related behaviors and behavioral consequences may be quite different. In this study, we focus mainly on the observation of locomotor hyperactivity to reveal underlying neurotransmitter changes. Due to the relative ease of quantification, locomotor activity testing has been widely used in modeling the positive symptoms of schizophrenia Van den Buuse and de Jong, 1989. On the other hand, cognitive impairments constitute a central feature of schizophrenia and, indeed, schizophrenic patients are commonly described to possess abnormalities in attention and information processing Tsai and Coyle, 2002. From observations of tests in the rats, it was clear that they exhibited schizophrenia-like symptoms after ketamine injection. The observations are in accordance with those reported by Olesen et al. (2012) & Hamon and Blier (2013) Hamon and Blier, 2013Olesen et al., 2012.
The present study also showed that treatment with QF or QFSLN, prior to ketamine injection, ameliorated ketamine-induced schizophrenic symptoms in rats. The effect of QFSLN was more pronounced. The original DA hypothesis states that hyperactive DA transmission results in schizophrenic symptoms. This hypothesis is the main theory related to the incidence of schizophrenic symptoms. The antipsychotic drug QF blocks DA transmission, thus alleviating the schizophrenic symptoms. Riedel et al. (2007) reported that QF is a dibenzothiazepine derivative with a relatively broad receptor binding profile. It has major affinity to cerebral serotonergic, histaminergic and dopaminergic D1 and D2 receptors, moderate affinity to adrenergic receptors 1 and 2, and minor affinity to muscarinergic M1 receptor Riedel et al., 2007. The efficacy of QF in reducing the symptoms of schizophrenia has been demonstrated in several clinical trials; in these trials, QF showed efficacy in treating some of the aggressive symptoms. In accordance with our results, Duncan et al. (1998) reported that pre-treatment of rats with QF blocked metabolic activation in the brain induced by ketamine Duncan et al., 1998.
To date, the mechanism of action of anti-psychotic drugs in schizophrenia have been linked to DA and its interaction with other neurochemicals including glutamate, GABA, 5-HT and acetylcholine Redrobe et al., 2012. GABA and glutamate regulate the activity of DA. The activation of GABA receptors in the brain results in various receptor interactions with glutamate and modulation of GABA receptors improves cognitive deficits in rats. Maeshima et al. (2007) concluded that the atypical anti-psychotic drug QF electively binds to central DA D2 and 5-HT receptors, leading to reduction in the level of DA and symptoms of schizophrenia Maeshima et al., 2007. Some theories suggest that the neurotransmitter serotonin may also play a role in causing the symptoms of schizophrenia Riedel et al., 2007. QF antipsychotic drug can treat these symptoms by blocking DA and serotonin transmissions.
Since the antipsychotic drug QF has poor oral bioavailability (about 9% only) Sweetman, 2007, it is important to find ways to increase its absorption and bioavailability; the use of SLN is one of these approaches. In this study we succeeded in generating QFSLN, with particle size ranging from 134-162 nm. Narala and Veerabrahma (2013), similarly, generated QFSLN with mean size of 200 nm (and entrapment efficiency between 80-92%) Narala and Veerabrahma, 2013; they discovered that the relative bioavailability was increased by 3.7 fold compared to that for QF. In general, delivering QF to the brain is a great challenge due to the blood brain barrier and, therefore, SLN formulation has been exploited as a potential drug carrier for tissue targeting. In addition, SLN are composed of solid lipid matrix that provides an effective means for controlled release of the drugs. It also improves drug bioavailability in the brain.
Thus, our present study was designed to enhance the bioavailability of QF in the brain by incorporating a minimal dose of QF into SLN, which act as a carrier Parvanthi et al., 2014. In addition, Rathi et al. (2013) showed that preparations of QFSLN were capable of promoting sustained drug release over a period of 48 h Rathi, 2013. This may reduce the concentration of the drug to be administered along with frequency of dosing, thereby minimizing the occurrence of side effects, improve bioavailability and increase the effectiveness of the drug. With regard to the effects of QF and QFSLN on hepatorenal function, CK and lipid patterns were evaluated after 3 weeks of treatment. The results showed that there was a slight increase in hepatorenal function and lipid parameters in the QF treatment group. Since the dose of nanoparticles used in this study was the same dose as QF suspension, this suggests that the bioavailability of QFSLN was markedly increased. These results are in agreement with those reported by Goldstein (1999), who described an increased activity of enzymes, particularly of ALT Goldstein, 1999. Meyer and Koro (2004) demonstrated that during QF treatment, the level of serum cholesterol and triglycerides were slightly elevated Meyer and Koro, 2004. The slight effect of QF on hepatorenal function and lipid profile in the present study might be due to QF treatment, which is essential for optimal therapeutic response. Adaptation induced by the drug exposure could provide novel treatment strategies that would mimic or promote adaptive responses. In some preclinical models, different effects have been seen after chronic (compared to acute) antipsychotic administration. For example, acute treatment with atypical antipsychotic drugs enhance NMDA-induced electrophysiological responses but chronic treatment with drugs reduce NMDA receptor sensitivity Yang and Reis, 1999.
Moreover, the results of the immunohistochemical assay showed negative or low Bcl2 expression after ketamine induction. Previous studies have shown that ketamine induces neuronal apoptosis through down-regulation of Bcl2 expression Hardwick and Soane, 2013. The groups of rats treated with QF and QFSLN showed an increase of Bcl2 expression in the cortex and hippocampus of the brain tissue. Bcl2 is known to inhibit apoptosis by preventing the release of cytochrome C and the subsequent activation of caspases Hardwick and Soane, 2013. Thus, the beneficial effects of QF and its nanoparticle form (QFSLN) on the aforementioned behavioral and biochemical assays may also be related to their potential effects on neuroprotection and/or neurogenesis beyond the DA and 5-HT receptor blockade effects Lodge and Grace, 2011.
Conclusion
Ketamine induction caused schizophrenic-like symptoms in rats. QF-loaded nanoparticles (QFSLN) was more effective than QF in improving the ketamine-induced schizophrenic-like symptoms in rats. The effect for both QF and QFSLN was dose-dependent. Thus, the nanoparticle form of QF showed increased solubility and enhanced efficacy as an antipsychotic drug. The use of QF-loaded nanoparticles may be beneficial for schizophrenia and perhaps other related diseases.
Abbreviations
5-HT: Serotonin
ALP: Alkaline Phosphatase
ALT: Alanine Aminotransferase
AST: Aspartate Aminotransferase
CK: Creatine Kinase
DA: Dopamine
GABA: Gamma-Aminobutyric Acid
GGT: Gamma-Glutamyl Transferase
HDL: High-Density Lipoprotein
LDL: Low-Density Lipoprotein
NE: Norepinephrine
QF: Quetiapine Fumarate
QFSLN: Quetiapine Fumarate Solid Lipid Nanoparticles
S.Alb: Serum Albumin
S.Alb/Glob: Serum Albumin/Globulin Ratio
S.Glob: Serum Globulin
S.T.P.: Serum Total Protein
Author Contribution
Gehan A. Elmenoufy performed behavioral tests, neurotransmitter, and amino acids analyses; Said M. M. performed the assessment of liver and kidney functions.
References
-
J.M.
Adams,
S.
Cory.
Life-or-death decisions by the Bcl-2 protein family. Trends in biochemical sciences.
2001;
26
:
61-66
.
-
D.
Arion,
T.
Unger,
D.A.
Lewis,
P.
Levitt,
K.
Mirnics.
Molecular evidence for increased expression of genes related to immune and chaperone function in the prefrontal cortex in schizophrenia. Biological psychiatry.
2007;
62
:
711-721
.
-
P.B.
Armitage,
JNS
G Matthews.
Comparison of several groups. In Statistical methods in medical research. Oxford: Blackwell Science Ltd.
2008;
:
208-235
.
-
D.M.
Barch,
C.S.
Carter,
T.S.
Braver,
F.W.
Sabb,
A.
MacDonald,
D.C.
Noll,
J.D.
Cohen.
Selective deficits in prefrontal cortex function in medication-naive patients with schizophrenia. Archives of general psychiatry.
2001;
58
:
280-288
.
-
S.
Bellino,
E.
Paradiso,
F.
Bogetto.
Efficacy and tolerability of quetiapine in the treatment of borderline personality disorder: A pilot study. The Journal of clinical psychiatry.
2006;
67
:
1042-1046
.
-
P.
Blasi,
A.
Schoubben,
G.
Traina,
G.
Manfroni,
L.
Barberini,
P.F.
Alberti,
C.
Cirotto,
M.
Ricci.
Lipid nanoparticles for brain targeting III. Long-term stability and in vivo toxicity. International journal of pharmaceutics.
2013;
454
:
316-323
.
-
L.
De Oliveira,
D.B.
Fraga,
R.D.
De Luca,
L.
Canever,
F.V.
Ghedim,
M.P.P.
Matos,
E.L.
Streck,
J.
Quevedo,
A.I.
Zugno.
Behavioral changes and mitochondrial dysfunction in a rat model of schizophrenia induced by ketamine. Metabolic brain disease.
2011;
26
:
69-77
.
-
G.E.
Duncan,
J.N.
Leipzig,
R.B.
Mailman,
J.A.
Lieberman.
Differential effects of clozapine and haloperidol on ketamine-induced brain metabolic activation. Brain research.
1998;
812
:
65-75
.
-
S.
El-Sisi,
S.
El-Nabarawy,
O.
Radwan,
A.
Abdel-Razek.
Comparative Study of Some Natural and Artificial Food Coloring Agents on Hyperactivity, Learning and Memory Performance in Weanling Rats. In International Journal of Sciences: Basic and Applied Research (IJSBAR) (North America).
2015
.
-
J.
Goldstein.
Quetiapine fumarate (Seroquel): a new atypical antipsychotic. Drugs of today.
1999;
(Barcelona
:
Spain: 1998) 35, 193-210
.
-
M.
Hamon,
P.
Blier.
Monoamine neurocircuitry in depression and strategies for new treatments. Progress in Neuro-Psychopharmacology and Biological Psychiatry.
2013;
45
:
54-63
.
-
J.M.
Hardwick,
L.
Soane.
Multiple functions of BCL-2 family proteins. Cold Spring Harbor perspectives in biology.
2013;
5
:
a008722
.
-
R.L.
Heinrikson,
S.C.
Meredith.
Amino acid analysis by reverse-phase high-p e r f o r m a n c e l i q u i d c h ro m at o g ra p h y : p re c o l u m n d e r i v at i z at i o n w i t h phenylisothiocyanate. Analytical biochemistry.
1984;
136
:
65-74
.
-
J.
Kreuter.
Nanoparticulate systems for brain delivery of drugs. Advanced drug delivery reviews.
2001;
47
:
65-81
.
-
J.H.
Krystal,
L.P.
Karper,
J.P.
Seibyl,
G.K.
Freeman,
R.
Delaney,
J.D.
Bremner,
G.R.
Heninger,
M.B.
Bowers,
D.S.
Charney.
Subanesthetic effects of the noncompetitive NMDA antagonist, ketamine, in humans: psychotomimetic, perceptual, cognitive, and neuroendocrine responses. Archives of general psychiatry.
1994;
51
:
199-214
.
-
A.S.
Levey,
J.
Coresh,
T.
Greene,
J.
Marsh,
L.A.
Stevens,
J.W.
Kusek,
F.
Van Lente.
Expressing the Modification of Diet in Renal Disease Study equation for estimating glomerular filtration rate with standardized serum creatinine values. Clinical chemistry.
2007;
53
:
766-772
.
-
D.J.
Lodge,
A.A.
Grace.
Developmental pathology, dopamine, stress and schizophrenia. International Journal of Developmental Neuroscience.
2011;
29
:
207-213
.
-
H.
Maeshima,
T.
Ohnuma,
Y.
Sakai,
N.
Shibata,
H.
Baba,
H.
Ihara,
M.
Higashi,
T.
Ohkubo,
E.
Nozawa,
S.
Abe.
Increased plasma glutamate by antipsychotic medication and its relationship to glutaminase 1 and 2 genotypes in schizophrenia—Juntendo University Schizophrenia Projects (JUSP). Progress in Neuro-Psychopharmacology and Biological Psychiatry.
2007;
31
:
1410-1418
.
-
A.K.
Malhotra,
D.A.
Pinals,
C.M.
Adler,
I.
Elman,
A.
Clifton,
D.
Pickar,
A.
Breier.
Ketamine-induced exacerbation of psychotic symptoms and cognitive impairment in neuroleptic-free schizophrenics. Neuropsychopharmacology.
1997;
17
:
141-150
.
-
A.K.
Malhotra,
D.A.
Pinals,
H.
Weingartner,
K.
Sirocco,
C.D.
Missar,
D.
Pickar,
A.
Breier.
NMDA receptor function and human cognition: the effects of ketamine in healthy volunteers. Neuropsychopharmacology.
1996;
14
:
301-307
.
-
R.L.
McLamb,
L.R.
Williams,
K.P.
Nanry,
W.A.
Wilson,
H.A.
Tilson.
MK-801 impedes the acquisition of a spatial memory task in rats. Pharmacology Biochemistry and Behavior.
1990;
37
:
41-45
.
-
W.
Mehnert,
K.
Mäder.
Solid lipid nanoparticles: production, characterization and applications. Advanced drug delivery reviews.
2001;
47
:
165-196
.
-
J.M.
Meyer,
C.E.
Koro.
The effects of antipsychotic therapy on serum lipids: a comprehensive review. Schizophrenia research.
2004;
70
:
1-17
.
-
R.H.
MuÈller,
K.
MaÈder,
S.
Gohla.
Solid lipid nanoparticles (SLN) for controlled drug delivery-a review of the state of the art. European journal of pharmaceutics and biopharmaceutics.
2000;
50
:
161-177
.
-
A.
Narala,
K.
Veerabrahma.
Preparation, characterization and evaluation of quetiapine fumarate solid lipid nanoparticles to improve the oral bioavailability. Journal of pharmaceutics.
2013;
2013
.
-
J.
Olesen,
A.
Gustavsson,
M.
Svensson,
H.U.
Wittchen,
B.
Jönsson.
The economic cost of brain disorders in Europe. European journal of neurology.
2012;
19
:
155-162
.
-
P.
Pagel,
J.
Blome,
H.U.
Wolf.
High-performance liquid chromatographic separation and measurement of various biogenic compounds possibly involved in the pathomechanism of Parkinson’s disease. Journal of Chromatography B: Biomedical Sciences and Applications.
2000;
746
:
297-304
.
-
N.N.C.E.P.E.
Panel.
Third report of the MCEP. Evaluation and treatment of high blood cholesterol in adults. Bethesda National Heart, lung and blood institute.
2001
.
-
M.
Parvanthi,
A.
Prathyusha,
R.
Kiran,
J.R.
Reddy.
Preparation and evaluation of quetiapine fumarate microemulsions: A novel delivery system. Asian J Pharm Clin Res.
2014;
7
:
208-213
.
-
B.S.
Pickard.
Schizophrenia biomarkers: translating the descriptive into the diagnostic. Journal of Psychopharmacology.
2015;
29
:
138-143
.
-
M.G.
Rathi,
AK Sachinkumar
S; Seth.
Development and characterization of quetiapine looded chitosan nanoparticle. Pharma Science Monitor.
2013;
4
:
150-169
.
-
J.P.
Redrobe,
L.
Elster,
K.
Frederiksen,
C.
Bundgaard,
I.E.
de Jong,
G.P.
Smith,
A.T.
Bruun,
P.H.
Larsen,
M.
Didriksen.
Negative modulation of GABAA α5 receptors by RO4938581 attenuates discrete sub-chronic and early postnatal phencyclidine (PCP)-induced cognitive deficits in rats. Psychopharmacology.
2012;
221
:
451-468
.
-
M.
Riedel,
N.
Müller,
M.
Strassnig,
I.
Spellmann,
E.
Severus,
H.-J.
Möller.
Quetiapine in the treatment of schizophrenia and related disorders. Neuropsychiatric disease and treatment.
2007;
3
:
219
.
-
G.
Schumann,
F.
Canalias,
J.
Joergensen Poul,
D.
Kang,
J.-M.
Lessinger,
R.
Klauke,
E.
on behalf of the Committee on Reference Systems for.
IFCC reference procedures for measurement of the catalytic concentrations of enzymes: corrigendum, notes and useful advice. In Clinical Chemistry and Laboratory Medicine.
2010;
615
.
-
S.
Sweetman.
Martindle, the complete Drug reference, 35 edn. London: Pharmaceutical Press.
2007
.
-
N.
Tietz.
Fundaments of Clinical Chemistry, 2 edn. 1994
.
-
N.
Tietz.
Clinical guide to laboratory tests, 3 edn (AACC). 1995
.
-
N.
Tietz.
The text book of clinical chemistry and molecular diagnosis (4th edn) Burtis. Ashwood and Bruns (Eds) Elsevier Saunders.
2005;
2290
.
-
G.
Tsai,
J.T.
Coyle.
Glutamatergic mechanisms in schizophrenia. Annual review of pharmacology and toxicology.
2002;
42
:
165-179
.
-
M.
Van den Buuse,
W.
de Jong.
Differential effects of dopaminergic drugs on open-field behavior of spontaneously hypertensive rats and normotensive Wistar-Kyoto rats. Journal of Pharmacology and Experimental Therapeutics.
1989;
248
:
1189-1196
.
-
Y.-C.
Wang,
U.-C.
Ho,
M.-C.
Ko,
C.-C.
Liao,
L.-J.
Lee.
Differential neuronal changes in medial prefrontal cortex, basolateral amygdala and nucleus accumbens after postweaning social isolation. Brain Structure and Function.
2012;
217
:
337-351
.
-
X.-C.
Yang,
D.J.
Reis.
Agmatine selectively blocks then-methyl-d-aspartate subclass of glutamate receptor channels in rat hippocampal neurons. Journal of Pharmacology and Experimental Therapeutics.
1999;
288
:
544-549
.
Comments
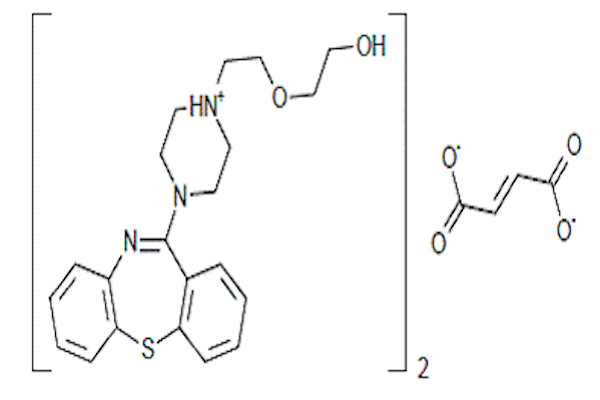
Downloads
Article Details
Volume & Issue : Vol 4 No 08 (2017)
Page No.: 1480-1497
Published on: 2017-08-07
Citations
Copyrights & License

This work is licensed under a Creative Commons Attribution 4.0 International License.
Search Panel
- HTML viewed - 6221 times
- Download PDF downloaded - 1740 times
- View Article downloaded - 9 times