Abstract
Stem cell therapy is a promising therapy for repairing damaged tissue. A growing body of research shows that stem cells work effectively in several diseases such as cardiovascular disease, hepatic disease, and diabetes. It has been shown that stem cells not only differentiate into functional cells and replace dead cells, but also release growth factors and cytokines which can recruit autologous cells. The most significant barrier to achieve clinical relevance of this treatment mode is the poor survival rate of injected cells. To improve transplantation and enhance functional outcome, investigations of gene transfection (overexpression of anti-apoptotic and antioxidant proteins), growth factor supplementation, and scaffolding matrices are being conducted. In this review, we will focus on methods to increase cell survival in stem cell transplantation as a novel treatment for cardiovascular disease.
Introduction
Cardiovascular disease is the leading cause of death globally. The World Health Organization (WHO) reported that about 17.5 million people died from this disease in 2012. Among many therapies and interventions that have been developed to treat the disease, stem cell therapy is one of the greatest potential candidates for treatment Fuster, 2014. For instance, unlike heart replacement, various sources of progenitor cells/stem cells are available - umbilical cord derived stem cells, bone marrow derived mesenchymal stem cells, adipose derived stem cells, embryonic stem cells, cardiac stem cells, and induced pluripotent stem cells. Therefore, it is possible to have a suitable cell type for a specific patient. Moreover, the therapy does not require a surgery, so it is safer, more affordable and requires less recovery time and resources. Cells can be injected into the heart by catheter or needle and syringe. Patients do not even need to undergo anesthesia. This can reduce morbidity and mortality in an already high-risk population. As a consequence, there have been several clinical trials using cell therapy to treat both acute myocardial infarction and chronic ischemia in human Fisher et al., 2014Fisher et al., 2015.
The heart lacks self-regeneration ability. After infarction, hypoxia-sensitive cardiomyocytes die and an inflammatory response ensues, removing the debris. Fibroblasts then proliferate and occupy the space, creating a collagenous, non-contractile scar which continues to remodel, causing negative effectives on cardiac function. The hope in using stem cells resides in the idea that they not only become heart cells, but also recruit autologous stem/progenitor cells, mobilized to the infarcted area, to recapitulate the highly-vascularized environment to support the metabolic demands. Additionally, many cytokines and chemokines are secreted to induce paracrine effects.
The goals are that cell therapy can repair the damaged heart by mediating some combination of the following mechanisms:
Differentiate to cardiomyocytes
Many stem cell types have been showed that they can express markers of cardiomyocytes and cardio-progenitor cells Boheler et al., 2002Quevedo et al., 2009Toma et al., 2002. For example, cardiomyocytes could be derived from embryonic stem cells when scientists cultivated embryoid body to day 7 and/or under the induction of several factors. When beating area appeared, they collected the cells from the population by enzyme (like collagenase) Laflamme et al., 2007Maltsev et al., 1993Maltsev et al., 1994. Then, they adjusted the expression of cardio-specific proteins like myosin heavy chain (MHC), α-actin, desmin, and cardiac troponin T Boheler et al., 2002. In addition to embryonic stem cells, messenchymal stem cells has also been studied the differentiation ability into cardiomyocytes both in vitro and in vivo Toma et al., 2002Xu et al., 2004. At the present, adipose tissue-derived stem cells (ADSC) and induced-pluripotent stem cells (iPSC) are the main focus. 5-azacytidine is used as an effective induced factor to start the differentiation process by activating transcription factor genes like GATA4, Nkx2.5 which then stimulate expression of cardiac-specific genes Burridge and Zambidis, 2013Carvalho et al., 2013Gherghiceanu et al., 2011Li et al., 2015. As a result of this potential, stem cell therapy has become a promising therapy to treat cardiovascular disease, especially diseases relevant to myocardial injury.
Release of paracrine factors
In addition to differential ability, transplanted stem cells also release paracrine signaling and recruit endogenous cardiac stem cells Gnecchi et al., 2008. A growing body of research has shown that the mechanism which stem cells can regenerate the damaged heart was the cells release soluble factors that stimulated the cardiac regeneration, neovascularization, and cardiac modeling after myocardial infarction Gnecchi et al., 2008Kinnaird et al., 2004Zhang et al., 2007. These factors could activate pro-survival signaling pathway like PI3K/Akt or STAT3 which protect cardiomyocytes and enhance cardiac regeneration Gnecchi et al., 2006. They contain factors such as bFGF, IGF-2, VEGF, IL-11. Additionally, these factors also recruit endogenous cardiac stem cells which, although the existence is controversial and if so, not present in significant numbers, have strong abilities to become heart cells, vascular smooth muscle cells, and endothelial cells in vivo Leri et al., 2005. These factors like IGF-1 and HGF released by transplanted Mesenchymal stem cells-MSCs were shown that they could activate endogenous cardiac stem cells Gnecchi et al., 2008. The broad spectrum of abilities of stem cells has been a significantly important issue that could explain the cardio-protection and remodeling of stem cell transplantation.
Cell fusion
Moreover, cell fusion between transplanted cells and domestic cells also has been used to explain the mechanism of damaged heart renewal by stem cell transplantation Doppler et al., 2013. Injected cells were demonstrated to fuse with host cardiomyocytes and stimulate the regeneration of injured hearts. These stem cell types were cardiac progenitor cells Oh et al., 2003, bone marrow stem cells Nygren et al., 2004, and adipose tissue-derived stem cells Metzele et al., 2011. This potential of stem cells has contributed to benefits of stem cell therapy for cardiovascular disease. Although stem cell transplantation is a promising therapy and has been under development for a long time since the first study was published Marelli et al., 1992, it also contains many challenges when cells are injected to hearts ( Figure 1 ). Firstly, poor cell survival caused by harsh environment in the infarcted area Zhang et al., 2001. When cells are transplanted to injured heart, they are immediately embedded in the ischemic condition which lacks oxygen, nutrients, and contain many apoptotic signals, causing an abundance of cell death as soon as the first hour after transplantation Haider and Ashraf, 2008Muller-Ehmsen et al., 2002Zhang et al., 2001. Secondly, cell engraftment has also been a major concern of stem cell therapy for heart disease. As a result of tenuous cell retention and cell survival, injected cells have difficulty integrating with the host tissue. The saline solution which is regularly used as a vehicle for delivery cannot keep the cells in the heart tissue because it does not contain a matrix for cell adherence of survival factors Li et al., 2016. More studies are needed to optimize the transplant conditions. To solve these two main problems in stem cell therapy for heart disease, scientists have suggested many solutions focusing on three approaches: gene transfection, growth factor introduction, and creation of hydrogel scaffolding ( Figure 2 ).
Gene transfection
Akt
Overexpressing Akt helps stem cells (like MSCs) exhibit a significantly enhanced intramyocardial retention and engraftment. It is believed that Akt is necessary and sufficient for cell survival because it not only targets apoptotic family members, but also increases glucose metabolism and releases energy during hypoxia Datta et al., 1999. In light of these advantages of Akt protein, Akt-modified MSCs genetically restore significantly greater myocardial volume than equal numbers of control cells Mangi et al., 2003. In addition to Akt signal transduction, many investigators work with hypoxia inducible factor 1 alpha (HIF-1α) and Pim-1 kinase.
HIF-1 alpha
HIF-1 alpha is a pro-angiogenic transcription factor which regulates the expression of many genes such as vascular endothelial growth factor (VEGF), angiopoietin 1 (ANGPT1) and ANGPT2, placental growth factor (PGF), and platelet-derived growth factor B (PDGFB) Dai et al., 2007Manalo et al., 2005. Under hypoxic conditions, hydroxyl reaction does not occur, causing HIF-1 protein to escape from inhibition and be activated, resulting in transcription of many genes and stimulates angiogenesis Pugh and Ratcliffe, 2003. Basing on the important function of HIF 1 in hypoxic cells, it’s expression was introduced in MSCs, which was expected that these MSCs could protect co-culture cardiomyocytes through HIF pathway Dai et al., 2007.
Pim-1
Pim-1 is a proto-oncogenic serine-threonine kinase which was first described in hematopoietic cells. The kinase is responsible for cell survival, proliferation, and differentiation in the cell type Wang et al., 2001. According to Aho et al, Pim-1 kinase inhibits pro-apototic Bad protein and increases Bcl-2 activity, promoting cell survival Aho et al., 2004. Recently, it is also demonstrated that Pim-1 plays an important role in cardioprotection downstream of Akt signaling Muraski et al., 2007. Fischer et al proves that Pim-1 overexpress – cardiac progenitor cells (Pim-1 – CPCs) survive and proliferate better than control CPCs Fischer et al., 2009. In this study, Pim-1-CPCs improve cardiac repair and regeneration through increasing in vascular density, cell proliferation, and persistence Fischer et al., 2009.
Protein
While genes must be introduced to cells before injection, growth factors can be supplied to the injected cells before or at the same time with transplantation. The following proteins have demonstrated promise as adjuncts to cellular therapy:
Ephrin
The Ephrin/Eph receptor tyrosine kinase (RTK) family is the largest of the RTK families. Unlike the other members of RTK family, Ephs specially can activated intracellular signals in both receptor exposing cells and ligand exposing cells that allows them to participate in many important biological processes such as development, proliferation, differentiation, and migration. There are two subclasses in Ephs family, including EphAs and EphBs that are segregated by their abilities to bind with the glycosylphosphatidylinositol-linked Ephrin-A ligands or the transmembrane-bound ephrin-B ligands. Typically, the receptors of specific groups respond to ligands of their corresponding groups , ie receptor EphA binds to ligand ephrinA. They are less or not bind to ligands of opposing groups , except ephrin B3 can attach and activate EphA4 and eprin A5 can bind and activate EphB2 O'Neal et al., 2013. Ephrin as a target for cardiovascular treatment was first described by Mansson-Broberg et al. in 2008 Mansson-Broberg et al., 2008. Since then, a few publications about the EphrinA/EphA-R signaling system in the heart have been produced Frieden et al., 2010Goichberg et al., 2011O'Neal et al., 2013. Goichberg et al showed that motility and migration of human cardiac stem cells (hCSCs) were enhanced by the increasing of interactions between EphA2 on hCSCs and ephrinA1 which presented on cardiomyocytes Goichberg et al., 2011. The Virag laboratory performed many studies to clarify the effects of this protein in cardio-protection after myocardial infarction Dries et al., 2011DuSablon et al., 2014O'Neal et al., 2014. They demonstrated that mice lacking EphA2 receptor expression caused negative effects on survival after myocardial infarction and made the remodeling process stagnant DuSablon et al., 2014. Furthermore, they demonstrated that EphrinA1 signaling could modulate inflammatory response and limit the infarct size O'Neal et al., 2014. Unlike cancer treatment Boyd et al., 2014, the approach using Ephrin signaling in heart disease treatment is still novel and more research is warranted to be assess clinical relevance.
Bone morphogenetic protein - BMP
BMP belongs to the transforming growth factor-beta (TGF-β) super family Chen et al., 2004. It is a key protein in mesoderm development and cardiac differentiation Chen et al., 2008. A growing body of research has shown that BMP is needed for pluripotent stem cells differentiating into cardiac cells. When supplied to the growth medium, BMP expands embryonic stem cells and has been demonstrated to promote cardiomyocyte differentiation Pal and Khanna, 2007. Using BMP4 and Activin A to induce ESCs to differentiate into cardiomyocytes, Laflamme et al presented a cocktail of pro-survival factors to enhance post-transplantation cell survival. This approach significantly improved ESC engraftment in myocardial infarction treatment. For example, it prevented death pathways to protect the cells on the transplanted grafts and helped the grafts survive 1 to 4 weeks after implantation. Therefore, the grafts could cause positive effects on the cardiac function like remuscularization, wall thickening, ventricular dilation decreasing, global function improving, and wall motion enhancement Behfar et al., 2002Laflamme et al., 2007. With the advantages, BMP should be researched to apply in cell therapy for heart disease treatment.
Fibroblast growth factor – FGF
More than 20 years ago, FGF was shown to be cardioprotective, especially after ischemia-reperfusion injury by Kardami et al. Kardami et al., 1993. FGF is a member of the family of heparin-binding growth factors and is involved in several important developmental processes Thisse and Thisse, 2005. In the heart, FGF works as cardioprotective and angiogenic agent Detillieux et al., 2003. It not only maintains cardiomyocyte survival, but also stimulates functional recovery after injury Rosenblatt-Velin et al., 2005, leading to the notion that using FGFs is a feasible approach to improve stem cell transplantation effectiveness. Recently, to pre-treat stem cells with FGF before transplantation, scientists combined it in a cocktail of growth factors such as TGF β, BMP-2, Activin A, etc. Behfar et al., 2010Hahn et al., 2008. In the future, FGFs might be applied in both stem cell culture and global tissue restoration after heart damaged.
Insulin-like growth factor 1–IGF-1
IGF-1 has been shown to reduce the doubling time of mesenchymal stem cells (MSC), improve MSC proliferation, and enhance CXCR4 expression Huang et al., 2012. IGF-1 also a demonstrated role in modulating cardiovascular function. It facilitates cardiac development, enhances cardiac contractility, and protects cardiac tissue after myocardial infarction Ren et al., 1999. Therefore, using IGF-1 accompany with transplanted cells has become a promising strategy. Davis et al. has reported that, when the combination of cardiomyocytes and IGF-1 in a “biotin sandwich” transplanted into injured hearts resulted in recovery of heart function Davis et al., 2006. This result suggested that IGF-1 could cause positive effects on both cardiac and injected cells and using IGF-1 in cell therapy needs further exploration.
Scaffold
To improve cell survival and cell engraftment in stem cell therapy for heart disease treatment, scaffolding to support cells is a valuable tool. Scaffolding helps us not only control the cell distribution, but also provide growth factors and structural support for transplanted cells.
Hydrogel
Although gene and growth factor strategies can improve cell survival, they do not contribute substantially to cell distribution and engraftment. To improve the stem cell transplantation effectiveness, a new approach called Matrix-Assisted Cell Transplantation (MACT) which creates a materials-based environment that enhances pro-survival paracrine signaling and then increase cell engraftment with the host tissues was born Parisi-Amon et al., 2013Prestwich, 2008. There are several materials that can be used in the strategy including natural and synthetic types like hydrogel, alginate, chitosan, collagen, etc. Benoit et al., 2008Kang et al., 2014. Subsequently, some scientists suggested that they will use hydrogel as a MACT to secrete growth factors and improve cell survival after transplantation Jha et al., 2015. Hyaluronic acid –based hydrogel is a promising candidate which contains key advantages such as biocompatibility, tunable properties, and native biofunctionality Highley et al., 2016Travan et al., 2016. It is wide used for biomedical applications, especially in heart disease treatment therapy Abdalla et al., 2013Bonafe et al., 2014Jha et al., 2015. More research is needed to validate its clinical potential as an adjunct to cellular engraftment therapies.
Natural heart scaffold
Decellularization of the heart is also an attractive and challenging approach. Scientists collect heart from deceased patients and used detergents like Triton-X-100 or SDS to decellularize the hearts. They pushed detergents through a tube to the heart's aorta to remove DNA, soluble protein, lipid, and other cellular material to perform a natural matrix. This matrix was then used to seed cells to create an engineered heart Ott et al., 2008. Finally, they transplant the heart to animal model Maher, 2013. The time and energy irequired to decellularize the tissue, seed the cells, grow the tissue to achieve functionality, and transplant it into an animal is an extremely labor- and time-intensive endeavor. However, “this train has left the station” and so there are several investigators that continue to focus on refining and expediting this strategy and the results are heartening Lu et al., 2013Tapias and Ott, 2014. Although it has tremendous challenges, it still holds remarkable advantages such as providing an excellent model to research cell development and differentiation in 3D, and improving our understanding of the complex, unique, and dynamic nature of the myocardial scaffolding. This strategy needs to be explored to optimize the decellulalrization process, which cell type(s) can be used, and the functional range of blood-pumping capacity.
Cell-derived matrices
Scaffold created from cell-derived matrices is also an attractive approach. Instead of using directly natural tissue (such as heart from the newly dead) to create scaffold, people used cells/stem cells to form matrices which mimicked natural extracellular matrices. Then, these cells would be removed. According to Fitzpatrick and McDevitt review, several approaches in using these scaffold for cardiovascular and other organs (like skeletal tissue or skin) regeneration have been conducted Fitzpatrick and McDevitt, 2015. For example, to perform heart valve or blood vessel, scientists cultured fibroblast or smooth muscle cells in sheets, then these sheets were wrapped around a mandrel to have a tubular structure Quint et al., 2012. Although there were several clinical trials using this strategy McAllister et al., 2009Quint et al., 2012Wystrychowski et al., 2011, it needs to be explored more about engineered cells and decellularization techniques Fitzpatrick and McDevitt, 2015 before applying on patients.
Conclusions and future directions
As stem cells provide a hopeful treatment for cardiovascular disease patients, despite controversial opinions about the potential of this therapy Abbott, 2014, there are numerous pre-clinical trials and clinical trials using them Kastrup et al., 2016Krishna et al., 2011Westerdahl et al., 2016. Our group has also reported that umbilical cord blood derived stem cells can differentiate into cardiac progenitor cells and that injection of induced cells into damaged heart reduced scar size, stabilized blood pressure, and maintained heart function Pham et al., 2015. Van der Spoel et al. has a systematic review and meta-analysis about stem cell therapy for ischemic heart disease in large animals van der Spoel et al., 2011. According to this review, stem cell therapy is safe and improves left ventricular ejection fraction (LVEF) after injury. Currently, Poulin and colleagues also have a similar review on 29 studies which showed that stem cell therapy could enhance LVEF, reduce infarcted size, and increase myocardial viability Poulin et al., 2016. In the future, stem cell therapy with its safety, effective, and affordable price will be a promising therapy to save heart disease patients and improve the quality of life. Improving the outcome of the therapy by enhancing transplanted cell survival, distribution, and engraftment need further modifications and refinements to ensure optimal conditions before they can be routinely applied.
Abbreviation
ADSC: Adipose tissue-derived stem cell; Akt: Serine/threonine-specific protein kinase; ANGPT1, 2: Angiopoietin 1, 2; Bcl-2: B-cell lymphoma 2; bFGF: Basic fibroblast growth factor; BMP: Bone morphogenetic protein; CXCR4: Chemokine receptor type 4; Ephs: Ephrins protein; FGF: Fibroblast growth factor; HGF: Hepatocyte growth factor; HIF: Hypoxia inducible factor; IGF-2: Insulin-like growth factor 2; IL-11: Interleukin 11: iPSC: induced-Pluripotent stem cell; LVEF: left ventricular ejection fraction; MACT: Matrix-Assisted Cell Transplantation; MHC: Myosin heavy chain; MSC: Mesenchymal stem cell: PDGFB: Platelet-derived growth factor B; PGF: Placental growth factor; PI3K: Phosphoinositide 3-kinase: RTK: The Ephrin/Eph receptor tyrosine kinase; STAT3: Signal transducer and activator of transcription 3; TGF-beta: Transforming growth factor beta; VEGF: vascular endothelial growth factor.
Author contribution
Anh Thi Van Bui created the outline and wrote the manuscript, Dr. Truc Le Buu Pham draw 2 figures and edited the manuscript, Assistant Professor Jitka Virag organized and edited the manuscript.
References
-
A.
Abbott.
Doubts over heart stem-cell therapy. Nature.
2014;
509
:
15-16
.
-
S.
Abdalla,
G.
Makhoul,
M.
Duong,
R.C.
Chiu,
R.
Cecere.
Hyaluronic acid-based hydrogel induces neovascularization and improves cardiac function in a rat model of myocardial infarction. Interactive cardiovascular and thoracic surgery.
2013;
17
:
767-772
.
-
T.L.
Aho,
J.
Sandholm,
K.J.
Peltola,
H.P.
Mankonen,
M.
Lilly,
P.J.
Koskinen.
Pim-1 kinase promotes inactivation of the pro-apoptotic Bad protein by phosphorylating it on the Ser112 gatekeeper site. FEBS letters.
2004;
571
:
43-49
.
-
A.
Behfar,
S.
Yamada,
R.
Crespo-Diaz,
J.J.
Nesbitt,
L.A.
Rowe,
C.
Perez-Terzic,
V.
Gaussin,
C.
Homsy,
J.
Bartunek,
A.
Terzic.
Guided cardiopoiesis enhances therapeutic benefit of bone marrow human mesenchymal stem cells in chronic myocardial infarction. Journal of the American College of Cardiology.
2010;
56
:
721-734
.
-
A.
Behfar,
L.V.
Zingman,
D.M.
Hodgson,
J.M.
Rauzier,
G.C.
Kane,
A.
Terzic,
M.
Puceat.
Stem cell differentiation requires a paracrine pathway in the heart. FASEB journal : official publication of the Federation of American Societies for Experimental Biology.
2002;
16
:
1558-1566
.
-
D.S.
Benoit,
M.P.
Schwartz,
A.R.
Durney,
K.S.
Anseth.
Small functional groups for controlled differentiation of hydrogel-encapsulated human mesenchymal stem cells. Nature materials.
2008;
7
:
816-823
.
-
K.R.
Boheler,
J.
Czyz,
D.
Tweedie,
H.T.
Yang,
S.V.
Anisimov,
A.M.
Wobus.
Differentiation of pluripotent embryonic stem cells into cardiomyocytes. Circulation research.
2002;
91
:
189-201
.
-
F.
Bonafe,
M.
Govoni,
E.
Giordano,
C.M.
Caldarera,
C.
Guarnieri,
C.
Muscari.
Hyaluronan and cardiac regeneration. Journal of biomedical science.
2014;
21
:
100
.
-
A.W.
Boyd,
P.F.
Bartlett,
M.
Lackmann.
Therapeutic targeting of EPH receptors and their ligands. Nat Rev Drug Discov.
2014;
13
:
39-62
.
-
P.W.
Burridge,
E.T.
Zambidis.
Highly efficient directed differentiation of human induced pluripotent stem cells into cardiomyocytes. Methods in molecular biology.
2013;
(Clifton
:
NJ) 997, 149-161
.
-
P.H.
Carvalho,
A.P.
Daibert,
B.S.
Monteiro,
B.S.
Okano,
J.L.
Carvalho,
D.N.
Cunha,
L.S.
Favarato,
V.G.
Pereira,
L.E.
Augusto,
R.J.
Del Carlo.
Differentiation of adipose tissue-derived mesenchymal stem cells into cardiomyocytes. Arquivos brasileiros de cardiologia.
2013;
100
:
82-89
.
-
D.
Chen,
M.
Zhao,
G.R.
Mundy.
Bone morphogenetic proteins. Growth factors.
2004;
(Chur
:
Switzerland) 22, 233-241
.
-
K.
Chen,
L.
Wu,
Z.Z.
Wang.
Extrinsic regulation of cardiomyocyte differentiation of embryonic stem cells. Journal of cellular biochemistry.
2008;
104
:
119-128
.
-
Y.
Dai,
M.
Xu,
Y.
Wang,
Z.
Pasha,
T.
Li,
M.
Ashraf.
HIF-1alpha induced-VEGF overexpression in bone marrow stem cells protects cardiomyocytes against ischemia. Journal of molecular and cellular cardiology.
2007;
42
:
1036-1044
.
-
S.R.
Datta,
A.
Brunet,
M.E.
Greenberg.
Cellular survival: a play in three Akts. Genes & development.
1999;
13
:
2905-2927
.
-
M.E.
Davis,
P.C.
Hsieh,
T.
Takahashi,
Q.
Song,
S.
Zhang,
R.D.
Kamm,
A.J.
Grodzinsky,
P.
Anversa,
R.T.
Lee.
Local myocardial insulin-like growth factor 1 (IGF-1) delivery with biotinylated peptide nanofibers improves cell therapy for myocardial infarction. Proceedings of the National Academy of Sciences of the United States of America.
2006;
103
:
8155-8160
.
-
K.A.
Detillieux,
F.
Sheikh,
E.
Kardami,
P.A.
Cattini.
Biological activities of fibroblast growth factor-2 in the adult myocardium. Cardiovascular research.
2003;
57
:
8-19
.
-
S.A.
Doppler,
M.A.
Deutsch,
R.
Lange,
M.
Krane.
Cardiac regeneration: current therapies-future concepts. Journal of thoracic disease.
2013;
5
:
683-697
.
-
J.L.
Dries,
S.D.
Kent,
J.A.
Virag.
Intramyocardial administration of chimeric ephrinA1-Fc promotes tissue salvage following myocardial infarction in mice. The Journal of physiology.
2011;
589
:
1725-1740
.
-
A.
DuSablon,
S.
Kent,
A.
Coburn,
J.
Virag.
EphA2-receptor deficiency exacerbates myocardial infarction and reduces survival in hyperglycemic mice. Cardiovascular diabetology.
2014;
13
:
114
.
-
K.M.
Fischer,
C.T.
Cottage,
W.
Wu,
S.
Din,
N.A.
Gude,
D.
Avitabile,
P.
Quijada,
B.L.
Collins,
J.
Fransioli,
M.A.
Sussman.
Enhancement of myocardial regeneration through genetic engineering of cardiac progenitor cells expressing Pim-1 kinase. Circulation.
2009;
120
:
2077-2087
.
-
S.A.
Fisher,
S.J.
Brunskill,
C.
Doree,
A.
Mathur,
D.P.
Taggart,
E.
Martin-Rendon.
Stem cell therapy for chronic ischaemic heart disease and congestive heart failure. The Cochrane database of systematic reviews.
2014;
4
:
Cd007888
.
-
S.A.
Fisher,
H.
Zhang,
C.
Doree,
A.
Mathur,
E.
Martin-Rendon.
Stem cell treatment for acute myocardial infarction. The Cochrane database of systematic reviews.
2015;
9
:
Cd006536
.
-
L.E.
Fitzpatrick,
T.C.
McDevitt.
Cell-derived matrices for tissue engineering and regenerative medicine applications. Biomaterials science.
2015;
3
:
12-24
.
-
L.A.
Frieden,
T.A.
Townsend,
D.B.
Vaught,
D.M.
Delaughter,
Y.
Hwang,
J.V.
Barnett,
J.
Chen.
Regulation of heart valve morphogenesis by Eph receptor ligand, ephrin-A1. Developmental dynamics : an official publication of the American Association of Anatomists.
2010;
239
:
3226-3234
.
-
V.
Fuster.
Top 10 cardiovascular therapies and interventions for the next decade. Nature reviews Cardiology.
2014;
11
:
671-683
.
-
M.
Gherghiceanu,
L.
Barad,
A.
Novak,
I.
Reiter,
J.
Itskovitz-Eldor,
O.
Binah,
L.M.
Popescu.
Cardiomyocytes derived from human embryonic and induced pluripotent stem cells: comparative ultrastructure. Journal of cellular and molecular medicine.
2011;
15
:
2539-2551
.
-
M.
Gnecchi,
H.
He,
N.
Noiseux,
O.D.
Liang,
L.
Zhang,
F.
Morello,
H.
Mu,
L.G.
Melo,
R.E.
Pratt,
J.S.
Ingwall.
Evidence supporting paracrine hypothesis for Akt-modified mesenchymal stem cell-mediated cardiac protection and functional improvement. FASEB journal : official publication of the Federation of American Societies for Experimental Biology.
2006;
20
:
661-669
.
-
M.
Gnecchi,
Z.
Zhang,
A.
Ni,
V.J.
Dzau.
Paracrine mechanisms in adult stem cell signaling and therapy. Circulation research.
2008;
103
:
1204-1219
.
-
P.
Goichberg,
Y.
Bai,
D.
D'Amario,
J.
Ferreira-Martins,
C.
Fiorini,
H.
Zheng,
S.
Signore,
F.
del Monte,
S.
Ottolenghi,
D.A.
D'Alessandro.
The ephrin A1-EphA2 system promotes cardiac stem cell migration after infarction. Circulation research.
2011;
108
:
1071-1083
.
-
J.Y.
Hahn,
H.J.
Cho,
H.J.
Kang,
T.S.
Kim,
M.H.
Kim,
J.H.
Chung,
J.W.
Bae,
B.H.
Oh,
Y.B.
Park,
H.S.
Kim.
Pre-treatment of mesenchymal stem cells with a combination of growth factors enhances gap junction formation, cytoprotective effect on cardiomyocytes, and therapeutic efficacy for myocardial infarction. Journal of the American College of Cardiology.
2008;
51
:
933-943
.
-
H.
Haider,
M.
Ashraf.
Strategies to promote donor cell survival: combining preconditioning approach with stem cell transplantation. Journal of molecular and cellular cardiology.
2008;
45
:
554-566
.
-
C.B.
Highley,
G.D.
Prestwich,
J.A.
Burdick.
Recent advances in hyaluronic acid hydrogels for biomedical applications. Current opinion in biotechnology.
2016;
40
:
35-40
.
-
Y.-l.
Huang,
R.-f.
Qiu,
W.-y.
Mai,
J.
Kuang,
X.-y.
Cai,
Y.-g.
Dong,
Y.-z.
Hu,
Y.-b.
Song,
A.-p.
Cai,
Z.-g.
Jiang.
Effects of insulin-like growth factor-1 on the properties of mesenchymal stem cells in vitro. Journal of Zhejiang University Science B.
2012;
13
:
20-28
.
-
A.K.
Jha,
K.M.
Tharp,
J.
Ye,
J.L.
Santiago-Ortiz,
W.M.
Jackson,
A.
Stahl,
D.V.
Schaffer,
Y.
Yeghiazarians,
K.E.
Healy.
Enhanced survival and engraftment of transplanted stem cells using growth factor sequestering hydrogels. Biomaterials.
2015;
47
:
1-12
.
-
H.
Kang,
C.
Wen,
Y.
Hwang,
Y.R.
Shih,
M.
Kar,
S.W.
Seo,
S.
Varghese.
Biomineralized matrix-assisted osteogenic differentiation of human embryonic stem cells. Journal of materials chemistry.
2014;
B
:
Materials for biology and medicine 2, 5676-5688
.
-
E.
Kardami,
R.R.
Padua,
K.B.S.
Pasumarthi,
L.
Liu,
B.W.
Doble,
S.E.
Davey,
P.A.
Cattini.
Basic fibroblast growth factor in cardiac myocytes: expression and effects. In Growth Factors and the Cardiovascular System, P. Cummins, ed.. Boston, MA: Springer US.
1993;
:
55-75
.
-
J.
Kastrup,
N.D.
Mygind,
A.A.
Qayyum,
A.B.
Mathiasen,
M.
Haack-Sorensen,
A.
Ekblond.
Mesenchymal stromal cell therapy in ischemic heart disease. Scandinavian cardiovascular journal : SCJ.
2016;
:
1-20
.
-
T.
Kinnaird,
E.
Stabile,
M.S.
Burnett,
C.W.
Lee,
S.
Barr,
S.
Fuchs,
S.E.
Epstein.
Marrow-derived stromal cells express genes encoding a broad spectrum of arteriogenic cytokines and promote in vitro and in vivo arteriogenesis through paracrine mechanisms. Circulation research.
2004;
94
:
678-685
.
-
K.A.
Krishna,
K.S.
Krishna,
R.
Berrocal,
K.S.
Rao,
K.R.S.
Sambasiva Rao.
Myocardial infarction and stem cells. Journal of Pharmacy and Bioallied Sciences.
2011;
3
:
182-188
.
-
M.A.
Laflamme,
K.Y.
Chen,
A.V.
Naumova,
V.
Muskheli,
J.A.
Fugate,
S.K.
Dupras,
H.
Reinecke,
C.
Xu,
M.
Hassanipour,
S.
Police.
Cardiomyocytes derived from human embryonic stem cells in pro-survival factors enhance function of infarcted rat hearts. Nature biotechnology.
2007;
25
:
1015-1024
.
-
A.
Leri,
J.
Kajstura,
P.
Anversa.
Cardiac stem cells and mechanisms of myocardial regeneration. Physiological reviews.
2005;
85
:
1373-1416
.
-
Q.
Li,
Z.K.
Guo,
Y.Q.
Chang,
X.
Yu,
C.X.
Li,
H.
Li.
Gata4, Tbx5 and Baf60c induce differentiation of adipose tissue-derived mesenchymal stem cells into beating cardiomyocytes. The international journal of biochemistry & cell biology.
2015;
66
:
30-36
.
-
X.
Li,
K.
Tamama,
X.
Xie,
J.
Guan.
Improving Cell Engraftment in Cardiac Stem Cell Therapy. Stem cells international.
2016;
2016
:
7168797
.
-
T.Y.
Lu,
B.
Lin,
J.
Kim,
M.
Sullivan,
K.
Tobita,
G.
Salama,
L.
Yang.
Repopulation of decellularized mouse heart with human induced pluripotent stem cell-derived cardiovascular progenitor cells. Nature communications.
2013;
4
:
2307
.
-
B.
Maher.
Tissue engineering: How to build a heart. Nature.
2013;
499
:
20-22
.
-
V.A.
Maltsev,
J.
Rohwedel,
J.
Hescheler,
A.M.
Wobus.
Embryonic stem cells differentiate in vitro into cardiomyocytes representing sinusnodal, atrial and ventricular cell types. Mechanisms of development.
1993;
44
:
41-50
.
-
V.A.
Maltsev,
A.M.
Wobus,
J.
Rohwedel,
M.
Bader,
J.
Hescheler.
Cardiomyocytes differentiated in vitro from embryonic stem cells developmentally express cardiac-specific genes and ionic currents. Circulation research.
1994;
75
:
233-244
.
-
D.J.
Manalo,
A.
Rowan,
T.
Lavoie,
L.
Natarajan,
B.D.
Kelly,
S.Q.
Ye,
J.G.
Garcia,
G.L.
Semenza.
Transcriptional regulation of vascular endothelial cell responses to hypoxia by HIF-1. Blood.
2005;
105
:
659-669
.
-
A.A.
Mangi,
N.
Noiseux,
D.
Kong,
H.
He,
M.
Rezvani,
J.S.
Ingwall,
V.J.
Dzau.
Mesenchymal stem cells modified with Akt prevent remodeling and restore performance of infarcted hearts. Nature medicine.
2003;
9
:
1195-1201
.
-
A.
Mansson-Broberg,
A.J.
Siddiqui,
M.
Genander,
K.H.
Grinnemo,
X.
Hao,
A.B.
Andersson,
E.
Wardell,
C.
Sylven,
M.
Corbascio.
Modulation of ephrinB2 leads to increased angiogenesis in ischemic myocardium and endothelial cell proliferation. Biochemical and biophysical research communications.
2008;
373
:
355-359
.
-
D.
Marelli,
C.
Desrosiers,
M.
el-Alfy,
R.L.
Kao,
R.C.
Chiu.
Cell transplantation for myocardial repair: an experimental approach. Cell transplantation.
1992;
1
:
383-390
.
-
T.N.
McAllister,
M.
Maruszewski,
S.A.
Garrido,
W.
Wystrychowski,
N.
Dusserre,
A.
Marini,
K.
Zagalski,
A.
Fiorillo,
H.
Avila,
X.
Manglano.
Effectiveness of haemodialysis access with an autologous tissue-engineered vascular graft: a multicentre cohort study. Lancet.
2009;
(London
:
England) 373, 1440-1446
.
-
R.
Metzele,
C.
Alt,
X.
Bai,
Y.
Yan,
Z.
Zhang,
Z.
Pan,
M.
Coleman,
J.
Vykoukal,
Y.H.
Song,
E.
Alt.
Human adipose tissue-derived stem cells exhibit proliferation potential and spontaneous rhythmic contraction after fusion with neonatal rat cardiomyocytes. FASEB journal : official publication of the Federation of American Societies for Experimental Biology.
2011;
25
:
830-839
.
-
J.
Muller-Ehmsen,
P.
Whittaker,
R.A.
Kloner,
J.S.
Dow,
T.
Sakoda,
T.I.
Long,
P.W.
Laird,
L.
Kedes.
Survival and development of neonatal rat cardiomyocytes transplanted into adult myocardium. Journal of molecular and cellular cardiology.
2002;
34
:
107-116
.
-
J.A.
Muraski,
M.
Rota,
Y.
Misao,
J.
Fransioli,
C.
Cottage,
N.
Gude,
G.
Esposito,
F.
Delucchi,
M.
Arcarese,
R.
Alvarez.
Pim-1 regulates cardiomyocyte survival downstream of Akt. Nature medicine.
2007;
13
:
1467-1475
.
-
J.M.
Nygren,
S.
Jovinge,
M.
Breitbach,
P.
Sawen,
W.
Roll,
J.
Hescheler,
J.
Taneera,
B.K.
Fleischmann,
S.E.
Jacobsen.
Bone marrow-derived hematopoietic cells generate cardiomyocytes at a low frequency through cell fusion, but not transdifferentiation. Nature medicine.
2004;
10
:
494-501
.
-
W.T.
O'Neal,
W.F.
Griffin,
J.L.
Dries-Devlin,
S.D.
Kent,
J.
Chen,
M.S.
Willis,
J.A.
Virag.
Ephrin-Eph signaling as a potential therapeutic target for the treatment of myocardial infarction. Medical hypotheses.
2013;
80
:
738-744
.
-
W.T.
O'Neal,
W.F.
Griffin,
S.D.
Kent,
F.
Faiz,
J.
Hodges,
J.
Vuncannon,
J.A.
Virag.
Deletion of the EphA2 receptor exacerbates myocardial injury and the progression of ischemic cardiomyopathy. Frontiers in physiology.
2014;
5
:
132
.
-
H.
Oh,
S.B.
Bradfute,
T.D.
Gallardo,
T.
Nakamura,
V.
Gaussin,
Y.
Mishina,
J.
Pocius,
L.H.
Michael,
R.R.
Behringer,
D.J.
Garry.
Cardiac progenitor cells from adult myocardium: homing, differentiation, and fusion after infarction. Proceedings of the National Academy of Sciences of the United States of America.
2003;
100
:
12313-12318
.
-
H.C.
Ott,
T.S.
Matthiesen,
S.K.
Goh,
L.D.
Black,
S.M.
Kren,
T.I.
Netoff,
D.A.
Taylor.
Perfusion-decellularized matrix: using nature's platform to engineer a bioartificial heart. Nature medicine.
2008;
14
:
213-221
.
-
R.
Pal,
A.
Khanna.
Similar pattern in cardiac differentiation of human embryonic stem cell lines, BG01V and ReliCellhES1, under low serum concentration supplemented with bone morphogenetic protein-2. Differentiation; research in biological diversity.
2007;
75
:
112-122
.
-
A.
Parisi-Amon,
W.
Mulyasasmita,
C.
Chung,
S.C.
Heilshorn.
Protein-engineered injectable hydrogel to improve retention of transplanted adipose-derived stem cells. Advanced healthcare materials.
2013;
2
:
428-432
.
-
T.
Pham,
T.T.
Nguyen,
A.
Bui,
T.H.
Pham,
K.N.
Phan,
M.
Nguyen,
P.
Pham.
Preliminary evaluation of treatment efficacy of umbilical cord blood-derived mesenchymal stem cell-differentiated cardiac progenitor cells in a myocardial injury mouse model. Biomedical Research and Therapy.
2015;
2
:
1-11
.
-
M.F.
Poulin,
A.
Deka,
B.
Mohamedali,
G.L.
Schaer.
Clinical Benefits of Stem Cells for Chronic Symptomatic Systolic Heart Failure A Systematic Review of the Existing Data and Ongoing Trials. Cell transplantation.
2016
.
-
G.D.
Prestwich.
Engineering a clinically-useful matrix for cell therapy. Organogenesis.
2008;
4
:
42-47
.
-
C.W.
Pugh,
P.J.
Ratcliffe.
Regulation of angiogenesis by hypoxia: role of the HIF system. Nature medicine.
2003;
9
:
677-684
.
-
H.C.
Quevedo,
K.E.
Hatzistergos,
B.N.
Oskouei,
G.S.
Feigenbaum,
J.E.
Rodriguez,
D.
Valdes,
P.M.
Pattany,
J.P.
Zambrano,
Q.
Hu,
I.
McNiece.
Allogeneic mesenchymal stem cells restore cardiac function in chronic ischemic cardiomyopathy via trilineage differentiating capacity. Proceedings of the National Academy of Sciences of the United States of America.
2009;
106
:
14022-14027
.
-
C.
Quint,
M.
Arief,
A.
Muto,
A.
Dardik,
L.E.
Niklason.
Allogeneic human tissue-engineered blood vessel. Journal of vascular surgery.
2012;
55
:
790-798
.
-
J.
Ren,
W.K.
Samson,
J.R.
Sowers.
Insulin-like growth factor I as a cardiac hormone: physiological and pathophysiological implications in heart disease. Journal of molecular and cellular cardiology.
1999;
31
:
2049-2061
.
-
N.
Rosenblatt-Velin,
M.G.
Lepore,
C.
Cartoni,
F.
Beermann,
T.
Pedrazzini.
FGF-2 controls the differentiation of resident cardiac precursors into functional cardiomyocytes. Journal of Clinical Investigation.
2005;
115
:
1724-1733
.
-
L.F.
Tapias,
H.C.
Ott.
Decellularized Scaffolds as a Platform for Bioengineered Organs. Current opinion in organ transplantation.
2014;
19
:
145-152
.
-
B.
Thisse,
C.
Thisse.
Functions and regulations of fibroblast growth factor signaling during embryonic development. Developmental Biology.
2005;
287
:
390-402
.
-
C.
Toma,
M.F.
Pittenger,
K.S.
Cahill,
B.J.
Byrne,
P.D.
Kessler.
Human mesenchymal stem cells differentiate to a cardiomyocyte phenotype in the adult murine heart. Circulation.
2002;
105
:
93-98
.
-
A.
Travan,
F.
Scognamiglio,
M.
Borgogna,
E.
Marsich,
I.
Donati,
L.
Tarusha,
M.
Grassi,
S.
Paoletti.
Hyaluronan delivery by polymer demixing in polysaccharide-based hydrogels and membranes for biomedical applications. Carbohydrate polymers.
2016;
150
:
408-418
.
-
T.I.
Spoel,
S.J.
Jansen of Lorkeers,
P.
Agostoni,
E.
van Belle,
M.
Gyongyosi,
J.P.
Sluijter,
M.J.
Cramer,
P.A.
Doevendans,
S.A.
Chamuleau.
Human relevance of pre-clinical studies in stem cell therapy: systematic review and meta-analysis of large animal models of ischaemic heart disease. Cardiovascular research.
2011;
91
:
649-658
.
-
Z.
Wang,
N.
Bhattacharya,
M.
Weaver,
K.
Petersen,
M.
Meyer,
L.
Gapter,
N.S.
Magnuson.
Pim-1: a serine/threonine kinase with a role in cell survival, proliferation, differentiation and tumorigenesis. Journal of veterinary science.
2001;
2
:
167-179
.
-
D.E.
Westerdahl,
D.H.
Chang,
M.A.
Hamilton,
M.
Nakamura,
T.D.
Henry.
Allogeneic mesenchymal precursor cells (MPCs): an innovative approach to treating advanced heart failure. Expert opinion on biological therapy.
2016;
:
1-7
.
-
W.
Wystrychowski,
L.
Cierpka,
K.
Zagalski,
S.
Garrido,
N.
Dusserre,
S.
Radochonski,
T.N.
McAllister,
N.
L'Heureux.
Case study: first implantation of a frozen, devitalized tissue-engineered vascular graft for urgent hemodialysis access. The journal of vascular access.
2011;
12
:
67-70
.
-
W.
Xu,
X.
Zhang,
H.
Qian,
W.
Zhu,
X.
Sun,
J.
Hu,
H.
Zhou,
Y.
Chen.
Mesenchymal stem cells from adult human bone marrow differentiate into a cardiomyocyte phenotype in vitro. Experimental biology and medicine.
2004;
(Maywood
:
NJ) 229, 623-631
.
-
M.
Zhang,
N.
Mal,
M.
Kiedrowski,
M.
Chacko,
A.T.
Askari,
Z.B.
Popovic,
O.N.
Koc,
M.S.
Penn.
SDF-1 expression by mesenchymal stem cells results in trophic support of cardiac myocytes after myocardial infarction. FASEB journal : official publication of the Federation of American Societies for Experimental Biology.
2007;
21
:
3197-3207
.
-
M.
Zhang,
D.
Methot,
V.
Poppa,
Y.
Fujio,
K.
Walsh,
C.E.
Murry.
Cardiomyocyte grafting for cardiac repair: graft cell death and anti-death strategies. Journal of molecular and cellular cardiology.
2001;
33
:
907-921
.
Comments
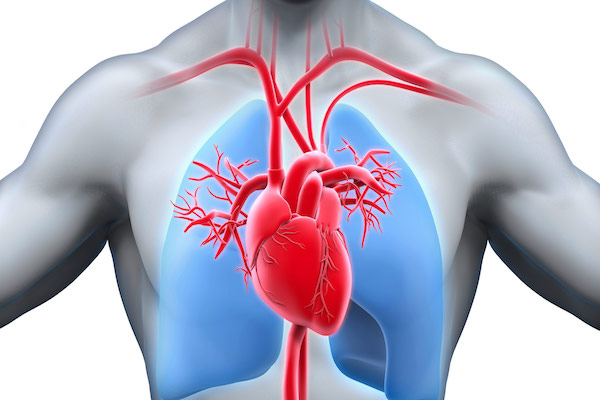
Downloads
Article Details
Volume & Issue : Vol 4 No 1 (2017)
Page No.: 1082-1097
Published on: 2017-01-17
Citations
Copyrights & License

This work is licensed under a Creative Commons Attribution 4.0 International License.
Search Panel
Pubmed
Google Scholar
Pubmed
Google Scholar
Pubmed
Search for this article in:
Google Scholar
Researchgate
- HTML viewed - 5960 times
- Download PDF downloaded - 1932 times
- View Article downloaded - 26 times