Abstract
Mesenchymal stem cells (MSCs) are the most widely used stem cells of the human body due to ease of successful isolation and expansion for many years. In particular, from 2012 until now, MSCs have been widely clinically used to treat various diseases, including graft versus host disease (GVHD), Crohn’s disease, and knee osteoarthritis. In this review, the applications of MSCs in diabetes will be reviewed and discussed. Diabetes mellitus type 1, also known as Type 1 diabetes (T1DM), is an autoimmune disease in which immune cells attack the beta cells in islets of Langerhans (pancreatic islets). Although type 2 diabetes (T2DM) is considered to be a disease related to insulin resistance, several recent studies have shown some relation of immune dysfunction in this disease. Therefore, MSC transplantation may be a beneficial treatment for both T1DM and T2DM. MSC transplantation in preclinical trials and clinical trials for T1DM and T2DM have shown a moderate to significant improvement in diabetes without adverse side effects. In this review, we will discuss some of the updates from preclinical and clinical trials of MSC transplantation for diabetes.
Introduction
Diabetes mellitus (DM) is a metabolic disorder caused by deficient insulin secretion or insulin dysfunction leading to hyperglycemia as well as chronic metabolic change of carbohydrates, protein and fat Bastaki, 2005. Chronic hyperglycemia leads to many serious complications, namely kidney failure, heart issue, and eye diseases. Thus, it considered a costly disease because a majority of countries spend 5% to 20% of their health expense on diabetes, according to the International Diabetes Federation (IDF) IDF, 2015. Moreover, diabetes is a high-incidence disease. In 2004, there were more than 200 million DM patients; the predicted prevalence of DM is expected to double by 2025 Halban, 2004. Also according to the IDF, of the approximate 7 billion people worldwide, about 415 million adults (aged 20-79) suffered from diabetes in 2015 and that number is expected to rise to 642 million people in 2040 IDF, 2015. The Western Pacific region of the world has the highest prevalence of diabetes, with 153 million cases which account for about 37% of total worldwide cases.
Diabetes is classified into two main types (type 1 and type 2) which both lead to hyperglycemia Moorefield, 2012. Diabetes mellitus type 1, or Type 1 diabetes (T1DM), is described as a genetic autoimmune disease. It is caused by a severe deficiency of insulin due to damage of pancreatic islet beta cells Zhang et al., 2008. T1DM patients have to depend on exogenous insulin injection to stabilize their blood glucose. Diabetes mellitus type 2 (T2DM) accounts for 90-95% of diabetes cases, and is caused by insulin resistance in peripheral tissues. T2DM is related to many risk factors, such as obesity, hypertension, lifestyle, age, family history, past history of impaired glucose tolerance (IGT) and impaired fasting glucose (IFG), and gestational diabetes (GDM). Many of the risk factors can be preventable.
Diabetic specialists have been studying ways to optimize therapy for diabetes since current therapies have two major limitations. The first is the challenge of insulin injection; there is long-term dependency, difficulty in adjusting the exact amount of exogenous insulin appropriate for each moment, and potential of insulin resistance when used long-term. The second limitation is pancreas/ islet/ islet cell transplantation; there is the problem of donor shortage and non-availability, potential of transplant rejection, and difficulty to functionally activate and prolong grafted materials. Therefore, improved treatments for diabetes which can address the aforementioned limitations will have valuable application to both diabetes research and management.
Recently, stem cell therapy has achieved positive results in medicine related to the 3Rs (Replacement, Repair and Regeneration). Of note, 3R therapy uses stem cells and progenitor cells as effective tools to prevent, repair, replace and treat damaged organs. In fact, stem cell therapy has been applied successfully in diabetes, from preclinical to clinical studies Dave et al., 2014Dong et al., 2008Gao et al., 2014Le et al., 2016Si et al., 2012Thi-Tung Dang, 2015Voltarelli et al., 2011Wu et al., 2015Wu et al., 2007Xiao et al., 2013Xie et al., 2016Yang et al., 2010Zhou et al., 2009. Stem cell transplantation is an excellent platform for diabetes therapy due to the fact that it can slow down the progression of diabetes and eliminate the complications of long-term blood glucose homeostatic effect.
The most popular stem cell type that has been evaluated in diabetes mellitus treatments have been mesenchymal stem cells (MSCs). These cells have great potential and it is feasible to isolate them, there is an abundant source, and ethical concerns are minimal. MSCs are isolated from various tissues, including adipose tissue, bone marrow, umbilical cord blood, umbilical cord and dental pulp Pham et al., 2014Ren et al., 2016Van Pham et al., 2016.
Mesenchymal stem cells
Mesenchymal stem cells (MSCs) have been demonstrated to be involved in the in vivo self-repair and self-regeneration processes of animal tissues. These cells can be isolated from different tissues such as bone marrow, adipose tissue, dental pulp, fetal appendages as well as umbilical cord blood, umbilical cord, and placenta. When cultured in vitro, they appear as spindle-shaped cells. MSCs express a specific marker profile; they are positive for CD29, CD51, CD73, CD90, and CD105 expression, yet negative for hematopoietic markers such as CD31 and CD45 Wang et al., 2014. Interestingly, they do not express MHC class II and only express MHC class I at low levels. Moreover, they do not express Fas ligand and costimulatory molecules such as B7 and CD40, thus they have been suggested to be hypoimmunogenic cells Atoui and Chiu, 2012. Other properties include their ability to self-renew, to create fibroblast colony forming units (CFU-F), and to differentiate into other cells such as bone, cartilage and fat. The aforementioned characteristics are part of criteria used to determine and identify MSCs Dominici et al., 2006.
Although the term “mesenchymal” defines their origin and differentiation tendency, MSCs are flexible and can change in vitro to exhibit features of specific cells or progenitor cells belonging to the endoderm or ectoderm layers. From the culmination of many protocols focused on optimizing their culture, at present it is feasible to efficiently isolate and expand MSCs in vitro and in vivo Ducret et al., 2016Pham et al., 2014Sensebe et al., 2011. Moreover, MSCs have been shown to play a role in the healing process in applications of MSC-based therapies for several diseases, such as heart dysfunction, neurodegenerative diseases, liver diseases, renal failure, and diabetes. Despite positive results of the therapy, it remains unclear as to the mechanisms exerted by MSCs. In this review, we will explore and discuss the role of MSCs in diabetic treatment.
Mesenchymal stem cells and diabetes mellitus
It is known that MSCs are active participants in the healing process of damaged tissues. Although they are present and can be isolated from diabetic human and animal tissues, the repair process is limited due to inadequate and impaired functions of MSCs Cianfarani et al., 2013Tobita et al., 2015. Adult MSCs can proliferate and differentiate in vitro by the effects of several soluble and non-soluble factors in the culture medium. A diabetic or hyperglycemic environment reduces the proliferative potential of MSCs yet increases senescence and apoptosis of the cells Cheng et al., 2016Cianfarani et al., 2013Cramer et al., 2010Hankamolsiri et al., 2016Kim et al., 2015Wajid et al., 2015. Moreover, the differentiation and immunomodulation capacity of diabetic or high glucose treated MSCs are distinct from normal cells Montanucci et al., 2016. A high glucose condition boosts adipogenic differentiation while decreasing osteogenic differentiation Cramer et al., 2010Hankamolsiri et al., 2016 and diminishing angiogenic capacity Kim et al., 2015 of affected MSCs.
Interestingly, a small proportion (6%) of diabetic MSCs express proinsulin and C-peptide and these cells can differentiate rapidly into functional islet-like cells in the presence of a pseudo diabetic milieu Phadnis et al., 2009. It has been suggested that the diabetic microenvironment may be a condition for MSC differentiation into insulin-producing cells in vitro and in vivo. Moreover, expression of stem cell specific surface markers on diabetic MSCs decreased Cianfarani et al., 2013 while expression of pluripotent markers increased Cheng et al., 2016. Additionally, hyperglycemia conditioned MSCs showed a reduction of cell migration Cheng et al., 2016Cianfarani et al., 2013 and of secretion of growth factors related to wound healing Cianfarani et al., 2013. Therefore, the reduced expression of certain important features, such as the above, warrants more investigations in MSC applications for diabetes treatment.
Mesenchymal stem cell transplantation for diabetes mellitus
It is known that MSCs play a crucial role in healing damaged tissues. They can differentiate to replace the dead cells as well as secrete stimulant factors to activate surrounding cells in the microenvironment, enhancing the tissue repair process Wang et al., 2014. Therefore, MSCs can be applied to treat tissues impaired by chronic hyperglycemia. For T1DM, MSC transplantation can theoretically increase beta cell mass via the following effects:
(1) beta cell replacement through in vitro or in vivo differentiation;
(2) local microenvironment modification by production of cytokines, chemokines and factors to stimulate endogenous regeneration;
(3) reduction or prevention of autoimmunity to beta cells Ezquer, 2014. Although several MSC transplantation studies have clearly shown the outcome of controlled glucose metabolism, there have been observations of decreased insulin resistance as well as enhanced beta cell function effects. Moreover, the mechanisms of MSC treatment for T2DM still has not been well understood. Some studies have suggested that the immunomodulatory and inflammatory effects of MSCs are what contribute to the resulting reduction of insulin resistance Liu et al., 2014Xie et al., 2016.
Safety of human mesenchymal stem cell transplantation
The first concern of any therapy is the risk of mortality. Similar to modern generation drugs, MSC-based therapies should be controlled and monitored for safety before their positive effects are determined. In diabetic animal models, graft rejection as well as acute adverse responses or sudden death were not noticed after xenograft MSC and/or differentiated MSC transplantations Gabr et al., 2013Ho et al., 2012Hu et al., 2015Lee et al., 2006Xie et al., 2016. Interestingly, graft tolerance was observed when human cells were transplanted
into diabetic mice without immunosuppressive treatment Chao et al., 2008Zhou et al., 2015. Moreover, MSC treated diabetic rodents survived and showed a prolonged life relative to non-treated diabetic animals Kadam et al., 2010Tsai et al., 2015. MSC transplantation was proven to be safe and well-tolerated in a small cohort of T2DM patients although approximately 22% of them had slight transient fevers Kong et al., 2014.
Efficacy of human mesenchymal stem cell transplantation
Preclinical treatment
Many studies have shown that human MSCs (hMSCs) or/and hMSC-derived islet-like cells effectively safety transplanted into diabetic animals Ho et al., 2012Kim et al., 2012 ( Table 1 ).
Human MSC transplantations lead to alleviated blood glucose levels in diabetic animals. It was confirmed that hMSC infusion could improve blood glucose homeostasis in both type 1 and type 2 diabetic animals. The glucose levels decreased markedly from a few days to 2 weeks after hMSC infusion and this decrease was maintained until 20 days to 10 weeks thereafter Ammar et al., 2015Ho et al., 2012Hu et al., 2014Hu et al., 2015Kadam et al., 2010Lee et al., 2006Tsai et al., 2015Xie et al., 2016Yang et al., 2010Zhou et al., 2015.
Multiple transplantations of hMSCs could improve the effects of hyperglycemia Ho et al., 2012Lee et al., 2006. Glucose levels were notably normalized after seven administrations of hMSCs Ho et al., 2012.
Other research studies have shown the efficiency of hMSC derived insulin-producing cells (IPCs) on diabetic treatments. These cells could be in vitro differentiated from many sources of hMSCs and exhibited many characteristics of actual beta cells, including C-peptide expression, insulin production, glucose response ability as well as pancreatic beta cell specific gene expression Gabr et al., 2013Kao et al., 2015Kim et al., 2015Seyedi et al., 2015Seyedi et al., 2016Thi-Tung Dang, 2015Van Pham et al., 2014Zhang and Dou, 2014. The IPCs were transplanted into liver Chao et al., 2008, renal capsule Hu et al., 2009Kadam et al., 2010; blood glucose levels were reduced 3 days thereafter and normalized at more than 9 weeks out or until removal of graft Chao et al., 2008Kadam et al., 2010Zhang et al., 2010. However, some results have shown that hIPC transplantations does not lower glucose levels Hu et al., 2009Ngoc et al., 2011.
Clinical applications
Although many preclinical studies have shown evidence that hMSC therapy has beneficial effects in the treatment of diabetes mellitus, there are still not many clinical applications of MSC therapy for T1DM or T2DM in the world. The first problem is due to the safety issue of MSC transplantation ( Table 2 ). However, this can be alleviated by understanding the special characteristics as well as in vitro and in vivo behavior of MSCs through experimental studies and evidence. MSCs themselves show unchanged morphology and phenotype as well as the normal karyotype. They also express tumor suppressors and oncogenes at normal levels even after they undergo long-term culture. There is no evidence that tumor formation is associated with MSC transplantation. Additionally, most of the clinical trials have proven that MSC transplantation for the treatment of diabetes is safe although there have been a few reported cases of fever. However, it is difficult to elucidate whether the fever was caused by the cell transplantation or from diabetic symptoms or certain infections.
The second problem or issue is whether MSC therapy is effective for the treatment of DM in humans. Although the mechanism has not been clearly demonstrated, MSC transplantation is capable of reducing blood glucose in various periods of follow-up time from a few months to several years. Moreover, it has been suggested that MSC transplantation can normalize or maintain the ameliorated blood glucose levels as well as improve serum insulin levels, C-peptide, HbA1C, and the daily insulin requirement for a long period. Beside evidence of these systemic effects, MSC transplantation has the potential to treat diabetic complications such as foot ulcers, thrombosis, heart failure, kidney failure, and blindness. Therefore, it is meaningful that MSC therapy can be applied to treat early and/or late stages of diabetes as well as relieve the pain of complications and delay or cease the need for amputations. Moreover, based on the successful clinical trials, many MSC therapies continue to be developed to improve the efficiencies of the following:
(1) prolonged time effect;
(2) reduced expenditure on treatment and increase in patients treated; and
(3) upgrade in cell products, from abundance and availability (commercial distribution) to development of diverse sources of MSCs and improvement of identifiable therapeutic characteristics of MSCs (e.g. immunomodulatory potential).
Moreover, MSCs have been suggested as universal therapeutic cells Atoui and Chiu, 2012 based on their immune privilege and are being developed as ready-to-use products (e.g. Prochymal). Finally, the mechanisms related to MSC therapy, while still unclear, are gradually being discovered through more research findings. As of now, pertinent questions remain such as:
(i) how do MSCs survive,
(ii) how do MSCs behave in live bodies, and
(iii) what reactions and responses occur after cell transplantation over time. Therefore, studies of MSCs and their application for the treatment of diabetes are valuable to understand the underlying mechanisms.
What role do hMSCs play after they are administrated in diabetic bodies?
Survival
Labeled MSCs have been transplanted into diabetic mice to determine their survival in body tissues. It was confirmed that the grafted cells presented and secreted functional human insulin and C-peptide Xin et al., 2016Zhang et al., 2010. Hess et al. found approximately 2.5% insulin-positive cells in diabetic mice whereas the cells were absent in the normal pancreas Hess et al., 2003. Lack of certain surface antigens on MSCs may result in a lowered immune responses in recipients as described above (safety issue).
Differentiation potential and cell replacement
It has been shown that MSC transplantation can ameliorate blood glucose levels within the follow-up time from few weeks to several years. However, the underlying mechanism of this effect is still unclear. One proposed mechanism is the replacement potential of transplanted MSC-derived cells. MSCs are able to trans-differentiate into insulin-producing cells and have been suggested to compensate impaired beta cells in diabetic animals. It is necessary to carefully determine the surrogate capability of MSCs. Although MSCs can be induced to secrete insulin in vitro and/or in vivo, few MSCs become fully functional beta cells in vivo (only approximately 1.7 – 3% of transplanted cells). Moreover, the transient survival of transfused cells confirms that the replacement potential may not be the only mechanism of the therapeutic effect of MSCs. Sordi et al. have suggested the role of MSCs as helper cells when they observed normalized blood glucose levels and neovascular formation after co-transplantation of pancreatic MSCs and islet mass Sordi et al., 2010.
Immune modulation - an important property of MSCs
Besides the differentiation potential, MSCs have the unique potential to modulate immune responses via several mechanisms. The in vitro immunosuppressive capability of MSCs was investigated since the late 1990s Wang et al., 2014. When co-cultured with leukocytes, it is found that MSCs are able to alter the proliferation of several immune cells. They are able to inhibit B lymphocytes Corcione et al., 2006, NK cells Spaggiari et al., 2008, inhibit differentiation and function of monocyte-derived dendritic cells Jiang et al., 2005, and suppress the activation and proliferation of T cells Nauta and Fibbe, 2007. These results have been confirmed by others Atoui and Chiu, 2012Mattar and Bieback, 2015Spaggiari et al., 2008Wang et al., 2014Yaochite et al., 2016Zhao et al., 2010. In particular, MSCs are able to induce macrophages to convert from proinflammatory type 1 to anti-inflammatory phenotype, resulting in alleviation of insulin resistance in T2DM Wang et al., 2014Xie et al., 2016. Moreover, it is specifically described that MSCs can alter the ratio of regulatory T cells versus other subtypes of T cells while capable of inducing the generation of regulatory T cells (Tregs) and simultaneously inhibiting the proliferation of T helper 1 (Th1) and Th17 cells Luz-Crawford et al., 2013. MSCs are capable of suppressing autoimmune responses in T1DM. Interestingly, these effects appear after direct interaction between MSCs and immune cells whereas the microenvironment components do not alter the immunomodulatory capability of MSCs in vitro Wehner et al., 2013.
It has been proposed that MSCs are not only multipotent adult stem cells but also universal donor cells due to their ability to avoid immune rejection Atoui and Chiu, 2012. Moreover, systemic administration of MSCs derived from autologous or allogeneic or even xenogeneic sources have been reported which can create non-specific systemic immunosuppression Nauta and Fibbe, 2007. The transplanted MSCs could survive and differentiate in allogeneic or xenogeneic recipients due to their immunotolerance capability. Atoui et al. have suggested that the underlying mechanism of immunotolerance capability of MSCs is due to their hypoimmunogenicity, T cell modulation and microenvironment immune suppression Atoui and Chiu, 2012. Consequently, transplanted MSCs can be tolerated (in part) in the recipients and can induce local pancreatic stem cells to proliferate, leading to replacement of impaired cells in the diabetic animals.
It has been found that MSCs can produce many cytokines and factors which improve and modulate the surrounding microenvironment. These components include inflammatory cytokines, immunosuppressive molecules and growth factors responsible for the tissue repair process Ma et al., 2014. The presence of inflammatory cytokines can initiate the immunomodulatory effects of MSCs via immunosuppressive factors such as nitric oxide (NO) in mice and indoleamine 2,3-dioxygenase (IDO) in humans, along with IL-10, TSG6, IL-6, LIF, PGE2, HO-1 and truncated CCL2 Ma et al., 2014. The immunomodulatory ability of MSCs is supposedly plastic since it depends on the inflammation status and cytokine status Ma et al., 2014Wang et al., 2014. Both NO and IDO play a role as a switch; strong inflammation drives MSCs to induce immunosuppression while weak inflammation enhances immune responses induced by MSCs Wang et al., 2014. Therefore, it is necessary to determine inflammation and cytokine levels as well as immunosuppressants to optimize the procedure of MSC transplantation.
Notably, it is perceived that MSCs derived from various tissues have different capabilities of immunomodulation Mattar and Bieback, 2015. This difference may derive from their origins or different culture conditions Menard et al., 2013.
Conclusion
Mesenchymal stem cells possess unique properties which make them suitable candidates for use in diabetes mellitus treatment strategies. Besides their potential to differentiate into various types of cells (e.g. beta cells), they also possess the ability to modulate immunity and angiogenesis via secreted paracrine factors. For Type 1 diabetes, the effects of MSCs on immune modulation are clearly evident; for Type 2 diabetes, some of the mechanisms exerted by MSCs are still unclear. However, for both T1DM and T2DM, MSC transplantation can dramatically improve the blood glucose levels, while reducing the insulin dose and side effects associated with DM. More importantly, both in preclinical trials and clinical trials, MSC transplantation has been demonstrated to be safe; there have been no observed adverse side effects or tumorigenesis. The data thus far have suggested that MSC transplantation is a promising therapy for DM. More controlled and randomized clinical trials are needed to further optimize the stem cell transplantation process.
Abbreviations
AMSC : Amniotic mesenchymal stem cell; BG: Blood glucose; BM: Bone Marrow; DPSC: Dental pulp stem cells from permanent teeth; DM: Diabetes mellitus; ESSC: Endometrial stromal stem cells; HFD: High fat diet; IPC: Insulin Producing Cell; MNC: Mononuclear Cell; MSC: Mesenchymal Stem Cell; NOD: Non-obese diabetic; PDPC: Periosteum-Derived Progenitor Cells; SCID: Severe Combined Immunodeficiency; SHED : Stem cells from pulps of human exfoliated deciduous teeth; STZ: Streptozotocin: T1DM: Type 1 Diabetes mellitus; T2DM: Type 2 Diabetes mellitus; UC: Umbilical Cord; UCB: Umbilical Cord Blood; WJMSC: Wharton’s Jelly Mesenchymal Stem Cell.
Author contribution
Ngoc Kim Phan has written the preclinical results showed in Table 1. Kiet Dinh Truong has written the clinical review. Loan Thi-Tung Dang has written the rest and completed and edited the review
References
-
H.I.
Ammar,
G.L.
Sequiera,
M.B.
Nashed,
R.I.
Ammar,
H.M.
Gabr,
H.E.
Elsayed,
N.
Sareen,
E.A.
Rub,
M.B.
Zickri,
S.
Dhingra.
Comparison of adipose tissue- and bone marrow- derived mesenchymal stem cells for alleviating doxorubicin-induced cardiac dysfunction in diabetic rats. Stem cell research & therapy.
2015;
6
:
148
.
-
R.
Atoui,
R.C.
Chiu.
Concise review: immunomodulatory properties of mesenchymal stem cells in cellular transplantation: update, controversies, and unknowns. Stem cells translational medicine.
2012;
1
:
200-205
.
-
S.
Bastaki.
Diabetes mellitus and its treatment. Int J Diabetes & Metabolism.
2005;
13
:
111-134
.
-
K.C.
Chao,
K.F.
Chao,
Y.S.
Fu,
S.H.
Liu.
Islet-like clusters derived from mesenchymal stem cells in Wharton's Jelly of the human umbilical cord for transplantation to control type 1 diabetes. PloS one.
2008;
3
:
e1451
.
-
N.C.
Cheng,
T.Y.
Hsieh,
H.S.
Lai,
T.H.
Young.
High glucose-induced reactive oxygen species generation promotes stemness in human adipose-derived stem cells. Cytotherapy.
2016;
18
:
371-383
.
-
F.
Cianfarani,
G.
Toietta,
G.
Di Rocco,
E.
Cesareo,
G.
Zambruno,
T.
Odorisio.
Diabetes impairs adipose tissue-derived stem cell function and efficiency in promoting wound healing. Wound repair and regeneration : official publication of the Wound Healing Society [and] the European Tissue Repair Society.
2013;
21
:
545-553
.
-
A.
Corcione,
F.
Benvenuto,
E.
Ferretti,
D.
Giunti,
V.
Cappiello,
F.
Cazzanti,
M.
Risso,
F.
Gualandi,
G.L.
Mancardi,
V.
Pistoia.
Human mesenchymal stem cells modulate B-cell functions. Blood.
2006;
107
:
367-372
.
-
C.
Cramer,
E.
Freisinger,
R.K.
Jones,
D.P.
Slakey,
C.L.
Dupin,
E.R.
Newsome,
E.U.
Alt,
R.
Izadpanah.
Persistent high glucose concentrations alter the regenerative potential of mesenchymal stem cells. Stem cells and development.
2010;
19
:
1875-1884
.
-
S.D.
Dave,
H.L.
Trivedi,
S.C.
Gopal,
T.
Chandra.
Combined therapy of insulin-producing cells and haematopoietic stem cells offers better diabetic control than only haematopoietic stem cells' infusion for patients with insulin-dependent diabetes. BMJ case reports 2014.
2014
.
-
M.
Dominici,
K.
Le Blanc,
I.
Mueller,
I.
Slaper-Cortenbach,
F.
Marini,
D.
Krause,
R.
Deans,
A.
Keating,
D.
Prockop,
E.
Horwitz.
Minimal criteria for defining multipotent mesenchymal stromal cells. The International Society for Cellular Therapy position statement. Cytotherapy.
2006;
8
:
315-317
.
-
Q.Y.
Dong,
L.
Chen,
G.Q.
Gao,
L.
Wang,
J.
Song,
B.
Chen,
Y.X.
Xu,
L.
Sun.
Allogeneic diabetic mesenchymal stem cells transplantation in streptozotocin-induced diabetic rat. Clinical and investigative medicine Medecine clinique et experimentale.
2008;
31
:
E328-337
.
-
M.
Ducret,
H.
Fabre,
O.
Degoult,
G.
Atzeni,
C.
McGuckin,
N.
Forraz,
F.
Mallein-Gerrin,
E.
Perrier-Groult,
J.C.
Fargues.
A standardized procedure to obtain mesenchymal stem/stromal cells from minimally manipulated dental pulp and Wharton's jelly samples. Bulletin du Groupement international pour la recherche scientifique en stomatologie & odontologie.
2016;
53
:
e37
.
-
M.
Ezquer.
Mesenchymal Stem Cell Therapy in Type 1 Diabetes Mellitus and Its Main Complications: From Experimental Findings to Clinical Practice. Journal of Stem Cell Research & Therapy.
2014;
04
.
-
M.M.
Gabr,
M.M.
Zakaria,
A.F.
Refaie,
A.M.
Ismail,
M.A.
Abou-El-Mahasen,
S.A.
Ashamallah,
S.M.
Khater,
S.M.
El-Halawani,
R.Y.
Ibrahim,
G.S.
Uin.
Insulin-producing cells from adult human bone marrow mesenchymal stem cells control streptozotocin-induced diabetes in nude mice. Cell transplantation.
2013;
22
:
133-145
.
-
X.
Gao,
L.
Song,
K.
Shen,
H.
Wang,
M.
Qian,
W.
Niu,
X.
Qin.
Bone marrow mesenchymal stem cells promote the repair of islets from diabetic mice through paracrine actions. Molecular and cellular endocrinology.
2014;
388
:
41-50
.
-
P.A.
Halban.
Cellular sources of new pancreatic beta cells and therapeutic implications for regenerative medicine. Nature cell biology.
2004;
6
:
1021-1025
.
-
W.
Hankamolsiri,
S.
Manochantr,
C.
Tantrawatpan,
D.
Tantikanlayaporn,
P.
Tapanadechopone,
P.
Kheolamai.
The Effects of High Glucose on Adipogenic and Osteogenic Differentiation of Gestational Tissue-Derived MSCs. Stem cells international.
2016;
2016
:
9674614
.
-
D.
Hess,
L.
Li,
M.
Martin,
S.
Sakano,
D.
Hill,
B.
Strutt,
S.
Thyssen,
D.A.
Gray,
M.
Bhatia.
Bone marrow-derived stem cells initiate pancreatic regeneration. Nature biotechnology.
2003;
21
:
763-770
.
-
J.H.
Ho,
T.C.
Tseng,
W.H.
Ma,
W.K.
Ong,
Y.F.
Chen,
M.H.
Chen,
M.W.
Lin,
C.Y.
Hong,
O.K.
Lee.
Multiple intravenous transplantations of mesenchymal stem cells effectively restore long-term blood glucose homeostasis by hepatic engraftment and beta-cell differentiation in streptozocin-induced diabetic mice. Cell transplantation.
2012;
21
:
997-1009
.
-
J.
Hu,
F.
Wang,
R.
Sun,
Z.
Wang,
X.
Yu,
L.
Wang,
H.
Gao,
W.
Zhao,
S.
Yan,
Y.
Wang.
Effect of combined therapy of human Wharton's jelly-derived mesenchymal stem cells from umbilical cord with sitagliptin in type 2 diabetic rats. Endocrine.
2014;
45
:
279-287
.
-
J.
Hu,
Y.
Wang,
F.
Wang,
L.
Wang,
X.
Yu,
R.
Sun,
Z.
Wang,
L.
Wang,
H.
Gao,
Z.
Fu.
Effect and mechanisms of human Wharton's jelly-derived mesenchymal stem cells on type 1 diabetes in NOD model. Endocrine.
2015;
48
:
124-134
.
-
Y.H.
Hu,
D.Q.
Wu,
F.
Gao,
G.D.
Li,
L.
Yao,
X.C.
Zhang.
A secretory function of human insulin-producing cells in vivo. Hepatobiliary & pancreatic diseases international : HBPD INT.
2009;
8
:
255-260
.
-
IDF.
. IDF Diabetes atlas Edition.
2015;
7
.
-
X.X.
Jiang,
Y.
Zhang,
B.
Liu,
S.X.
Zhang,
Y.
Wu,
X.D.
Yu,
N.
Mao.
Human mesenchymal stem cells inhibit differentiation and function of monocyte-derived dendritic cells. Blood.
2005;
105
:
4120-4126
.
-
S.S.
Kadam,
M.
Sudhakar,
P.D.
Nair,
R.R.
Bhonde.
Reversal of experimental diabetes in mice by transplantation of neo-islets generated from human amnion-derived mesenchymal stromal cells using immuno-isolatory macrocapsules. Cytotherapy.
2010;
12
:
982-991
.
-
S.Y.
Kao,
J.F.
Shyu,
H.S.
Wang,
C.H.
Lin,
C.H.
Su,
T.H.
Chen,
Z.C.
Weng,
P.J.
Tsai.
Comparisons of Differentiation Potential in Human Mesenchymal Stem Cells from Wharton's Jelly, Bone Marrow, and Pancreatic Tissues. Stem cells international.
2015;
2015
:
306158
.
-
H.
Kim,
J.W.
Han,
J.Y.
Lee,
Y.J.
Choi,
Y.D.
Sohn,
M.
Song,
Y.S.
Yoon.
Diabetic Mesenchymal Stem Cells Are Ineffective for Improving Limb Ischemia Due to Their Impaired Angiogenic Capability. Cell transplantation.
2015;
24
:
1571-1584
.
-
J.
Kim,
S.
Park,
H.M.
Kang,
C.W.
Ahn,
H.C.
Kwon,
J.H.
Song,
Y.J.
Lee,
K.H.
Lee,
H.
Yang,
S.Y.
Baek.
Human insulin secreted from insulinogenic xenograft restores normoglycemia in type 1 diabetic mice without immunosuppression. Cell transplantation.
2012;
21
:
2131-2147
.
-
D.
Kong,
X.
Zhuang,
D.
Wang,
H.
Qu,
Y.
Jiang,
X.
Li,
W.
Wu,
J.
Xiao,
X.
Liu,
J.
Liu.
Umbilical cord mesenchymal stem cell transfusion ameliorated hyperglycemia in patients with type 2 diabetes mellitus. Clinical laboratory.
2014;
60
:
1969-1976
.
-
P.T.-B.
Le,
P.
Van Pham,
N.B.
Vu,
L.T.-T.
Dang,
N.K.
Phan.
Expanded autologous adipose derived stem cell transplantation for type 2 diabetes mellitus. Biomedical Research and Therapy.
2016;
3
:
1034-1044
.
-
R.H.
Lee,
M.J.
Seo,
R.L.
Reger,
J.L.
Spees,
A.A.
Pulin,
S.D.
Olson,
D.J.
Prockop.
Multipotent stromal cells from human marrow home to and promote repair of pancreatic islets and renal glomeruli in diabetic NOD/scid mice. Proceedings of the National Academy of Sciences of the United States of America.
2006;
103
:
17438-17443
.
-
X.
Liu,
P.
Zheng,
X.
Wang,
G.
Dai,
H.
Cheng,
Z.
Zhang,
R.
Hua,
X.
Niu,
J.
Shi,
Y.
An.
A preliminary evaluation of efficacy and safety of Wharton's jelly mesenchymal stem cell transplantation in patients with type 2 diabetes mellitus. Stem cell research & therapy.
2014;
5
:
57
.
-
P.
Luz-Crawford,
M.
Kurte,
J.
Bravo-Alegria,
R.
Contreras,
E.
Nova-Lamperti,
G.
Tejedor,
D.
Noel,
C.
Jorgensen,
F.
Figueroa,
F.
Djouad.
Mesenchymal stem cells generate a CD4+CD25+Foxp3+ regulatory T cell population during the differentiation process of Th1 and Th17 cells. Stem cell research & therapy.
2013;
4
:
65
.
-
S.
Ma,
N.
Xie,
W.
Li,
B.
Yuan,
Y.
Shi,
Y.
Wang.
Immunobiology of mesenchymal stem cells. Cell death and differentiation.
2014;
21
:
216-225
.
-
P.
Mattar,
K.
Bieback.
Comparing the Immunomodulatory Properties of Bone Marrow, Adipose Tissue, and Birth-Associated Tissue Mesenchymal Stromal Cells. Frontiers in immunology.
2015;
6
:
560
.
-
C.
Menard,
L.
Pacelli,
G.
Bassi,
J.
Dulong,
F.
Bifari,
I.
Bezier,
J.
Zanoncello,
M.
Ricciardi,
M.
Latour,
P.
Bourin.
Clinical-grade mesenchymal stromal cells produced under various good manufacturing practice processes differ in their immunomodulatory properties: standardization of immune quality controls. Stem cells and development.
2013;
22
:
1789-1801
.
-
P.
Montanucci,
T.
Pescara,
I.
Pennoni,
A.
Alunno,
O.
Bistoni,
E.
Torlone,
G.
Luca,
R.
Gerli,
G.
Basta,
R.
Calafiore.
Functional Profiles of Human Umbilical Cord-Derived Adult Mesenchymal Stem Cells in Obese/Diabetic Versus Healthy Women. Current diabetes reviews.
2016
.
-
E.C.
Moorefield.
Stem cell-based regenerative pharmacology for the treatment of diabetes mellitus. 2012
.
-
A.J.
Nauta,
W.E.
Fibbe.
Immunomodulatory properties of mesenchymal stromal cells. Blood.
2007;
110
:
3499-3506
.
-
P.K.
Ngoc,
P.V.
Phuc,
T.H.
Nhung,
D.T.
Thuy,
N.T.
Nguyet.
Improving the efficacy of type 1 diabetes therapy by transplantation of immunoisolated insulin-producing cells. Human cell.
2011;
24
:
86-95
.
-
S.M.
Phadnis,
S.M.
Ghaskadbi,
A.A.
Hardikar,
R.R.
Bhonde.
Mesenchymal stem cells derived from bone marrow of diabetic patients portrait unique markers influenced by the diabetic microenvironment. The review of diabetic studies : RDS.
2009;
6
:
260-270
.
-
P.V.
Pham,
N.B.
Vu,
V.M.
Pham,
N.H.
Truong,
T.L.
Pham,
L.T.
Dang,
T.T.
Nguyen,
A.N.
Bui,
N.K.
Phan.
Good manufacturing practice-compliant isolation and culture of human umbilical cord blood-derived mesenchymal stem cells. Journal of translational medicine.
2014;
12
:
56
.
-
H.
Ren,
Y.
Sang,
F.
Zhang,
Z.
Liu,
N.
Qi,
Y.
Chen.
Comparative Analysis of Human Mesenchymal Stem Cells from Umbilical Cord, Dental Pulp, and Menstrual Blood as Sources for Cell Therapy. Stem cells international.
2016;
2016
:
3516574
.
-
L.
Sensebe,
P.
Bourin,
K.
Tarte.
Good manufacturing practices production of mesenchymal stem/stromal cells. Human gene therapy.
2011;
22
:
19-26
.
-
F.
Seyedi,
A.
Farsinejad,
M.
Moshrefi,
S.N.
Nematollahi-Mahani.
In vitro evaluation of different protocols for the induction of mesenchymal stem cells to insulin-producing cells. In vitro cellular & developmental biology Animal.
2015;
51
:
866-878
.
-
F.
Seyedi,
A.
Farsinejad,
S.A.
Nematollahi-Mahani,
T.
Eslaminejad,
S.N.
Nematollahi-Mahani.
Suspension Culture Alters Insulin Secretion in Induced Human Umbilical Cord Matrix-Derived Mesenchymal Cells. Cell journal.
2016;
18
:
52-61
.
-
Y.
Si,
Y.
Zhao,
H.
Hao,
J.
Liu,
Y.
Guo,
Y.
Mu,
J.
Shen,
Y.
Cheng,
X.
Fu,
W.
Han.
Infusion of mesenchymal stem cells ameliorates hyperglycemia in type 2 diabetic rats: identification of a novel role in improving insulin sensitivity. Diabetes.
2012;
61
:
1616-1625
.
-
V.
Sordi,
R.
Melzi,
A.
Mercalli,
R.
Formicola,
C.
Doglioni,
F.
Tiboni,
G.
Ferrari,
R.
Nano,
K.
Chwalek,
E.
Lammert.
Mesenchymal cells appearing in pancreatic tissue culture are bone marrow-derived stem cells with the capacity to improve transplanted islet function. Stem cells.
2010;
28
:
140-151
.
-
G.M.
Spaggiari,
A.
Capobianco,
H.
Abdelrazik,
F.
Becchetti,
M.C.
Mingari,
L.
Moretta.
Mesenchymal stem cells inhibit natural killer-cell proliferation, cytotoxicity, and cytokine production: role of indoleamine 2,3-dioxygenase and prostaglandin E2. Blood.
2008;
111
:
1327-1333
.
-
L.
Thi-Tung Dang,
A.
Nguyen-Tu Bui,
V.
Minh Pham,
N.
Kim Phan,
P.
Van Pham.
Production of islet-like insulin-producing cell clusters in vitro from adipose derived stem cells. Biomedical Research and Therapy.
2015;
2
:
184-192
.
-
M.
Tobita,
S.
Tajima,
H.
Mizuno.
Adipose tissue-derived mesenchymal stem cells and platelet-rich plasma: stem cell transplantation methods that enhance stemness. Stem cell research & therapy.
2015;
6
:
215
.
-
P.J.
Tsai,
H.S.
Wang,
G.J.
Lin,
S.C.
Chou,
T.H.
Chu,
W.T.
Chuan,
Y.J.
Lu,
Z.C.
Weng,
C.H.
Su,
P.S.
Hsieh.
Undifferentiated Wharton's Jelly Mesenchymal Stem Cell Transplantation Induces Insulin-Producing Cell Differentiation and Suppression of T-Cell-Mediated Autoimmunity in Nonobese Diabetic Mice. Cell transplantation.
2015;
24
:
1555-1570
.
-
P.
Van Pham,
P.
Thi-My Nguyen,
A.
Thai-Quynh Nguyen,
V.
Minh Pham,
A.
Nguyen-Tu Bui,
L.
Thi-Tung Dang,
K.
Gia Nguyen,
N.
Kim Phan.
Improved differentiation of umbilical cord blood-derived mesenchymal stem cells into insulin-producing cells by PDX-1 mRNA transfection. Differentiation; research in biological diversity.
2014;
87
:
200-208
.
-
P.
Van Pham,
N.B.
Vu,
N.K.
Phan.
Umbilical cord-derived stem cells (MODULATISTTM) show strong immunomodulation capacity compared to adipose tissue-derived or bone marrow-derived mesenchymal stem cells. Biomedical Research and Therapy.
2016;
3
:
687-696
.
-
J.C.
Voltarelli,
C.E.
Couri,
M.C.
Rodrigues,
D.A.
Moraes,
A.B.
Stracieri,
F.
Pieroni,
G.
Navarro,
A.M.
Leal,
B.P.
Simoes.
Stem cell therapies for type 1 diabetes mellitus. Indian journal of experimental biology.
2011;
49
:
395-400
.
-
N.
Wajid,
R.
Naseem,
S.S.
Anwar,
S.J.
Awan,
M.
Ali,
S.
Javed,
F.
Ali.
The effect of gestational diabetes on proliferation capacity and viability of human umbilical cord-derived stromal cells. Cell and tissue banking.
2015;
16
:
389-397
.
-
Y.
Wang,
X.
Chen,
W.
Cao,
Y.
Shi.
Plasticity of mesenchymal stem cells in immunomodulation: pathological and therapeutic implications. Nature immunology.
2014;
15
:
1009-1016
.
-
R.
Wehner,
C.
Taubert,
T.
Mende,
C.
Gaebler,
A.V.
de Andrade,
M.
Bornhauser,
C.
Werner,
T.
Tonn,
K.
Schakel,
M.
Bachmann.
Engineered extracellular matrix components do not alter the immunomodulatory properties of mesenchymal stromal cells in vitro. Journal of tissue engineering and regenerative medicine.
2013;
7
:
921-924
.
-
C.
Wu,
F.
Liu,
P.
Li,
G.
Zhao,
S.
Lan,
W.
Jiang,
X.
Meng,
L.
Tian,
G.
Li,
Y.
Li.
Engineered hair follicle mesenchymal stem cells overexpressing controlled-release insulin reverse hyperglycemia in mice with type L diabetes. Cell transplantation.
2015;
24
:
891-907
.
-
X.H.
Wu,
C.P.
Liu,
K.F.
Xu,
X.D.
Mao,
J.
Zhu,
J.J.
Jiang,
D.
Cui,
M.
Zhang,
Y.
Xu,
C.
Liu.
Reversal of hyperglycemia in diabetic rats by portal vein transplantation of islet-like cells generated from bone marrow mesenchymal stem cells. World journal of gastroenterology.
2007;
13
:
3342-3349
.
-
N.
Xiao,
X.
Zhao,
P.
Luo,
J.
Guo,
Q.
Zhao,
G.
Lu,
L.
Cheng.
Co-transplantation of mesenchymal stromal cells and cord blood cells in treatment of diabetes. Cytotherapy.
2013;
15
:
1374-1384
.
-
Z.
Xie,
H.
Hao,
C.
Tong,
Y.
Cheng,
J.
Liu,
Y.
Pang,
Y.
Si,
Y.
Guo,
L.
Zang,
Y.
Mu.
Human umbilical cord-derived mesenchymal stem cells elicit macrophages into an anti-inflammatory phenotype to alleviate insulin resistance in type 2 diabetic rats. Stem cells.
2016;
34
:
627-639
.
-
Y.
Xin,
X.
Jiang,
Y.
Wang,
X.
Su,
M.
Sun,
L.
Zhang,
Y.
Tan,
K.A.
Wintergerst,
Y.
Li,
Y.
Li.
Insulin-Producing Cells Differentiated from Human Bone Marrow Mesenchymal Stem Cells In Vitro Ameliorate Streptozotocin-Induced Diabetic Hyperglycemia. PloS one.
2016;
11
:
e0145838
.
-
Z.
Yang,
K.
Li,
X.
Yan,
F.
Dong,
C.
Zhao.
Amelioration of diabetic retinopathy by engrafted human adipose-derived mesenchymal stem cells in streptozotocin diabetic rats. Graefe's archive for clinical and experimental ophthalmology = Albrecht von Graefes Archiv fur klinische und experimentelle. Ophthalmologie.
2010;
248
:
1415-1422
.
-
J.N.
Yaochite,
K.W.
de Lima,
C.
Caliari-Oliveira,
P.V.
Palma,
C.E.
Couri,
B.P.
Simoes,
D.T.
Covas,
J.C.
Voltarelli,
M.C.
Oliveira,
E.A.
Donadi.
Multipotent mesenchymal stromal cells from patients with newly diagnosed type 1 diabetes mellitus exhibit preserved in vitro and in vivo immunomodulatory properties. Stem cell research & therapy.
2016;
7
:
14
.
-
L.
Zhang,
R.
Gianani,
M.
Nakayama,
E.
Liu,
M.
Kobayashi,
E.
Baschal,
L.
Yu,
S.
Babu,
A.
Dawson,
K.
Johnson.
Type 1 diabetes: chronic progressive autoimmune disease. Novartis Foundation symposium.
2008;
292
:
85-94; discussion 94
.
-
Y.
Zhang,
Z.
Dou.
Under a nonadherent state, bone marrow mesenchymal stem cells can be efficiently induced into functional islet-like cell clusters to normalize hyperglycemia in mice: a control study. Stem cell research & therapy.
2014;
5
:
66
.
-
Y.
Zhang,
W.
Shen,
J.
Hua,
A.
Lei,
C.
Lv,
H.
Wang,
C.
Yang,
Z.
Gao,
Z.
Dou.
Pancreatic islet-like clusters from bone marrow mesenchymal stem cells of human first-trimester abortus can cure streptozocin-induced mouse diabetes. Rejuvenation research.
2010;
13
:
695-706
.
-
S.
Zhao,
R.
Wehner,
M.
Bornhauser,
R.
Wassmuth,
M.
Bachmann,
M.
Schmitz.
Immunomodulatory properties of mesenchymal stromal cells and their therapeutic consequences for immune-mediated disorders. Stem cells and development.
2010;
19
:
607-614
.
-
H.
Zhou,
H.M.
Tian,
Y.
Long,
X.X.
Zhang,
L.
Zhong,
L.
Deng,
X.H.
Chen,
X.Q.
Li.
Mesenchymal stem cells transplantation mildly ameliorates experimental diabetic nephropathy in rats. Chinese medical journal.
2009;
122
:
2573-2579
.
-
Y.
Zhou,
Q.
Hu,
F.
Chen,
J.
Zhang,
J.
Guo,
H.
Wang,
J.
Gu,
L.
Ma,
G.
Ho.
Human umbilical cord matrix-derived stem cells exert trophic effects on beta-cell survival in diabetic rats and isolated islets. Disease models & mechanisms.
2015;
8
:
1625-1633
.
Comments
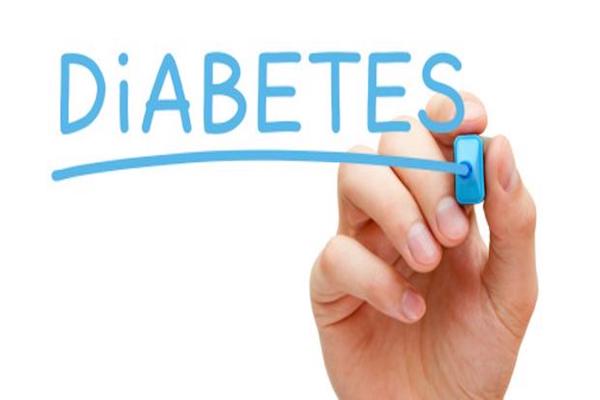
Downloads
Article Details
Volume & Issue : Vol 4 No 1 (2017)
Page No.: 1062-1081
Published on: 2017-01-15
Citations
Copyrights & License

This work is licensed under a Creative Commons Attribution 4.0 International License.
Search Panel
Pubmed
Google Scholar
Pubmed
Google Scholar
Pubmed
Search for this article in:
Google Scholar
Researchgate
- HTML viewed - 18049 times
- Download PDF downloaded - 4038 times
- View Article downloaded - 115 times