Abstract
Introduction: Type 2 diabetes mellitus (T2D) is the most common form of diabetes mellitus, accounting for 90% of diabetes mellitus in patients. At the present time, althoughT2D can be treated by various drugs and therapies using insulin replacement, reports have shown that complications including microvascular, macrovascular complications and therapy resistance can occur in patients on long term treatment. Stem cell therapy is regarded as a promising therapy for diabetes mellitus, including T2D. The aim of this study was to evaluate the safety and therapeutic effect of expanded autologous adipose derived stem cell (ADSC) transplantation for T2D treatment; the pilot study included 3 patients who were followed for 3 months.
Methods: The ADSCs were isolated from stromal vascular fractions, harvested from the belly of the patient,and expanded for 21 days per previously published studies. Before transplantation, ADSCs were evaluated for endotoxin, mycoplasma contamination, and karyotype.All patients were transfused with ADSCs at 1-2x106 cells/kg of body weight.Patients were evaluated for criteria related to transplantation safety and therapeutic effects; these included fever, blood glucose level before transplantation of ADSCs, and blood glucose level after transplantation (at 1, 2 and 3 months).
Results: The results showed that all samples of ADSCs exhibited the MSC phenotype with stable karyotype (2n=46), there was no contamination of mycoplasma, and endotoxin levels were low (<0.25 EU/mL). No adverse effects were detected after 3 months of transplantation. Decreases of blood glucose levels were recorded in all patients.
Conclusion: The findings from this initial study show that expanded autologous ADSCs may be a promising treatment for T2D.
Introduction
Diabetes mellitus (DM) affects approximately 300 million people worldwide Scully, 2012. DM and its complications cause substantial morbidity and mortality in DM patients. Islet transplantation have been used to treat DM with some promising results Hirshberg, 2007. Although these therapies can provide some benefit to patients there are limitations, namely the lack of pancreatic islets for transplantation Hirshberg, 2007McCall and Shapiro, 2012. In fact, two or three donors of pancreatic islets are required for a transplantation procedure for a single patient.
Recently, stem cell therapy has emerged as a highly promising new modality of treatment for advanced diabetes. For type 1 diabetes mellitus (T1D), both hematopoietic stem cell (HSC) transplantation and mesenchymal stem cell (MSC) transplantation have been evaluated Cantu-Rodriguez et al., 2016Cheng et al., 2016El-Badawy and El-Badri, 2016Snarski et al., 2016Xiang et al., 2016Xv et al., 2016. These studies have shown that HSC and MSC transplantations improve blood glucose levels from moderate to high. The meta-analysis study carried out by El-Badawy and El-Badri (2016) showed that the best therapeutic outcome could be achieved with CD34+ HSC transplantation, while the poorest outcome was observed with umbilical cord blood transplantation El-Badawy and El-Badri, 2016.
Stem cell therapy has also been used to treat type 2 diabetes mellitus (T2D). Tong et al. (2013) infused umbilical cord blood by microcatheter into the dorsal pancreatic artery in 3 T2D patients with different diabetic histories Tong et al., 2013. After the transplantation, Tong et al. showed that C-peptide levels increased in all of the patients; in fact reduced insulin dose corresponded with improved C-peptide levels Tong et al., 2013. In 2014, 22 patients with T2D were treated with Wharton’s jelly derived MSC (WJ-MSC) transplantation through intravenous injection and intra-pancreatic endovascular injection Liu et al., 2014. Liu et al. showed that WJ-MSC transplantation significantly decreased the levels of glucose, improved C-peptide levels, and improved beta cell function, all without any adverse effects Liu et al., 2014. In 2016, Hu et al. (2016) reported the long-term efficacy and safety of infusion of WJ-MSC on T2D in a study of61 patients with T2DM. After the 36-month follow-up period, infusion of WJ-MSC not only improved function of islet β-cells but reduced diabetic complications Hu et al., 2016.
Based on these results, we investigated the use of autologous expanded adipose derived stem cells (ADSCs) for transplantation to treat T2D patients. This study aimed to evaluate the preliminary outcome of autologous expanded ADSC transplantation, such as changes in blood glucose levels, at 1-3 months after transplantation.
Methods
Adipose tissue aspiration
Adipose tissues from patients were collected according to a previously published study Nguyen et al., 2016. Briefly, patients were given local anesthesia with 2-3 mL of marcaine (5 g/L); the lower abdomen area was also anesthetized. Patients were then given a tumescent solution (500 mL normal saline, 0.5 mL of 1:1000 epinephrine). A Triport Harvester cannula (Becton Dickinson, Franklin Lakes, NJ) and 60-cc BD Luer-Lock™ syringe (Becton Dickinson) were used for harvesting adipose tissues (100–500 cc) from patients. Isolation of stromal vascular fractions (SVFs) The SVFs were isolated from collected adipose tissues. Approximately 100 mL of lipoaspirate was collected from each patient into two 50 ml sterile syringes. The syringes were stored in a sterile box at 2–8°C and immediately transferred to the laboratory. The SVFs were isolated using a Cell Extraction Kit (RegenmedLab, Ho Chi Minh, Vietnam) according to the manufacturer’s instructions. Briefly, adipose tissues were digested using SuperDigest Solution (containing collagenase) included in the kit. The tissues were incubated in the solution at 37°C for 15 min with agitation at 5-min intervals. The suspension was centrifuged at 800×g for 10 min, and the SVFs were obtained as pellets. The pellet was washed twice with PBS to remove any residual enzyme, and re-suspended in PBS for determination of cell quantity and viability using an automatic cell counter (NucleoCounter; Chemometec, Lillerød, Denmark).
Activated platelet-rich plasma (PRP) preparation
Besides adipose tissue, activated PRP (aPRP) was prepared for each patient. PRP was isolated from peripheral blood using a kit (5PRP Kit, Regenmedlab, Ho Chi Minh, Vietnam) according to the manufacturer’s guidelines. Briefly, 20 mL of peripheral blood was collected into vacuum tubes and centrifuged at 800×g for 10 min. The plasma fraction was collected and centrifuged at 1000×g for 5 min to obtain a platelet pellet. Most of the plasma was then removed, leaving 6 mL of plasma for re-suspension of the platelets. The inactivated PRP was then activated using activating tubes containing 100 µL of 20% CaCl2.
Adipose derived stem cell culture
SVFs were cultured for expansion according to published procedures Van Pham et al., 2014. SVF samples were cultured in MSCCult medium (RegenMedLab, Ho Chi Minh, Vietnam) which contained DMEM/F12 supplemented with antibiotic and antimycotic solutions, 10 ng/mL epidermal growth factor (EGF), and 10 ng/mL basic fibroblast growth factor (bFGF) with 3% aPRP. The cells were plated at 5×104 cells/mL in T-75 flasks (Corning) and incubated at 37°C with 5% CO2. After 3 days of incubation, 6 mL of fresh medium was added to each flask. After 7 days, the medium was replaced with 12 mL of fresh medium. The culture medium was subsequently replaced every 3 days until the cells reached 70–80% confluence, at which point they were sub-cultured. The samples were cultured for 21 days with 3 sub-cultures.
Preparation of product for injection
The product for injection was composed of a mixture of the collected ADSCs and activated PRP. Activated PRP was used to dilute ADSCs to achieve a suitable dose for injection with 107 SVF cells/mL.
ADSC transplantation and monitoring
Patients were transfused with 106 ADSCs/kg in 250 mL of saline through the arm vein. Patients were monitored for blood glucose levels for every 2 weeks. Moreover, for longer evaluation, patients were monitored for C-peptide and HAb1c up to 12 months after transplantation.
Case representation
Case report: Case 1
Patient 1 (NTKO, female, year 1981) was diagnosed as T2D for 2 years. Patient 1 had adipose tissue at belly collected to isolate and culture ADSCs. After 21 days, the cells were confirmed to be MSCs since they were negative for CD14 and CD45, and positive forCD44 and CD90. The cells were evaluated and shown to have no mycoplasma contamination and low endotoxin (<0.25 EU/mL), as well as stable karyotype (2n=46). These cells were then harvested and re-suspended into 250 mL of physiology saline. The patient was transfused with her ADSCs in saline through the arm vein at a dose of 1x106 cells/kg for 30-45 min. Blood glucose was measured before transplantation and after transplantation (every 2 weeks until the report time). The results showed that the blood glucose level of Patient 1 gradually decreased after transplantation with her ADSCs, from 11.88 mM/L before transplantation to 9.85 mM/L, 10.89 mM/L, 9.49 mM/L and 7.84mM/L after 2, 4, 6, and 8 weeks of transplantation, respectively. There were no adverse effects recorded for Patient 1, especially hypoglycemia.
Case report: Case 2
Patient 2 (LTL, female, year 1959) was diagnosed with T2D for 2 years. Similar to patient 1, adipose tissue was also collected from the belly of the patient to isolate and culture ADSCs. The collected ADSCs also exhibited typical MSC phenotype, e.g. positive for CD44 and CD90, and negative for CD14 and CD45. The cells were negative for mycoplasma and showed low endotoxin level (<0.25 EU/mL). The cells were transfused to patient 2 at 1x106 cells/kg in 250 mL of saline for 30-45 min. No adverse effects was recorded in this patient during a 2-month follow up post transplantation. No attack of hypoglycemia was noted by patient and clinicians. Moreover, the blood glucose level gradually decreased from 8.04 mMl/L before transplantation to 7.05 mM/L and 7.07 mM/L after 2 weeks and 4 weeks of transplantation, respectively.
Case report: Case 3
Patient (DTL, female, year 1961) was confirmed as T2D for 2 years. The patient’s ADSCs were isolated and expanded for treatment. ADSCs were confirmed as MSCs from positive markers (CD44 and CD90) and they were also negative for CD14 and CD45. They also maintained the normal karyotype with 2n=46. These cells also were not contaminated with mycoplasma and had low endotoxin (<0.25 EU/mL). The cells were transplanted into the patient at a dose of 106 cells/kg in 250 mL of saline for 30 min. Patient 3 was monitored for 12 months. To report time, there was no side effects recorded for this patient. The transplantation caused a decrease of blood glucose level from 9.35 mM/L (before transplantation) to 7.32 mM/L (4 weeks post transplantation).
Discussion
T2D is complex disease with various mechanisms which likely induce insulin resistance in patients. In this study, we show that autologous expanded ADSC transplantation can reduce insulin resistance in the T2D patients. The mechanism by which ADSCs could reduce insulin resistance has been investigated in this study. However, based on the literature of several previous studies noting the effects of ADSCs and T2D pathophysiology, we recognized that there were likely connections between the two. The first clue showing the connection of ADSCs and T2D mechanism is chronic inflammation. There has been increasing evidence showing that T2D is linked to chronic inflammation which causes insulin resistance. Antuna-Puente et al. (2008) and Sell et al. (2012) showed that chronic inflammation of adipose tissue significantly contributed to insulin resistance via adipokines Antuna-Puente et al., 2008Sell et al., 2012.
The accumulation of macrophages in some tissues (e.g. vasculature, adipose tissue, muscle and liver) can cause the chronic metabolic stress-induced inflammation Bhargava and Lee, 2012. Moreover, macrophages play an important role in controlling the Th1/Th2 immune responses through the co-stimulating molecules CD80/CD86 and released cytokines Bhargava and Lee, 2012. These macrophages could produce inflammatory cytokines, such as IL-6 and TNFα, and induce insulin resistance in major metabolic tissues Bhargava and Lee, 2012Devaraj et al., 2010Rajwani et al., 2012.
These observations were confirmed in other previously published studies Kamei et al., 2006Kanda et al., 2006Patsouris et al., 2008. These studies showed that if depletion of CD11c+ macrophages or inhibition of macrophage recruitment via MCP-1 knockout in obese mice could significantly reduce inflammation and increase insulin sensitivity Kamei et al., 2006Kanda et al., 2006Patsouris et al., 2008. Increasing evidence in animals and humans about inflammation-induced insulin resistance in T2D were provided, and included abnormalities of lymphocytes DeFuria et al., 2013Winer et al., 2011, neutrophils Talukdar et al., 2012, eosinophils Wu et al., 2011, mast cells Liu et al., 2009 and dendritic cells Musilli et al., 2011. Therefore, the biggest challenge for treatment of T2D was modifying these immune cells to repair the insulin resistance.
ADSCs can participate in the correction of immune cells via their immune modulation capacity. In fact, while ADSCs can inhibit the proliferation of B cells, they can induce regulatory B cells Franquesa et al., 2015 and induce functional de-novo regulatory T cells with methylated FOXP3 gene DNA Engela et al., 2013. ADSCs can also successfully inhibit the allergic airway inflammation via immuno-modulation from a Th2 to a Th1-biased response in the mouse model Cho and Roh, 2010. Moreover, ADSCs can inhibit some chronic inflammatory diseases of the CNS Constantin et al., 2009.
ADSCs modulate the immune system via changes in anti-inflammatory cytokine expression and T-cell functions in hindlimb ischemia Kuo et al., 2011. By this mechanism, ADSC transplantation could protect the nephrotoxic injury resulting in reduced kidney damage and functional improvement, inhibiting organ fibrosis and providing long-term immune regulation Burgos-Silva et al., 2015Kim et al., 2012. By this mechanism, ADSCs have not only been successful at treating diseases in animal models but also in humans. Recently, Stepien et al. (2016) used ADSCs to treat Multiple Sclerosis in human; they showed that intrathecal treatment of ADSCs was a suitable therapy for cases with aggressive disease progression Stepien et al., 2016.
Hence, ADSC transplantation can modulate or correct the immune system, especially the chronic inflammation inside the T2D patients. Although, this report only introduces some results after 3 months follow up of 3 patients, the similar results after transplantation of ADSCs in all 3 patients shows that ADSC transplantation significantly reduces the insulin resistance.
In the previous clinical trials, using Wharton’s jelly derived MSCs, Liu et al. (2014) showed that MSC transplantation significantly decreased the levels of glucose and glycated hemoglobin, while improving C-peptide levels and beta cell function Liu et al., 2014. In another study, Jiang et al. (2011) used placenta derived MSCs to treat 10 T2D patients. The results showed that transplantation of MSC represents a simple, safe and effective therapeutic approach for T2D patients with islet cell dysfunction Jiang et al., 2011.
Although this investigation was a short study, with only evaluation of blood glucose levels, these initial results show that autologous expanded ADSC transplantation may be a promising therapy for T2D. This study has been modified and is now monitoring out to 12 months post transplantation; blood glucose levels as well as C-peptide and hemoglobin A1c (HbA1c) concentrations are being evaluated.
Conclusion
Our study shows some initial results of autologous expanded adipose derived stem cell transplantation for T2D. We show that this is a safe and effective therapy for T2D. In all 3 patient cases in our study, transplantation of autologous ADSCs gradually decreased blood glucose levels up to 2 months, without any adverse effects or complications. Moreover, ADSCs could successfully be isolated and could proliferate during expansion. They showed normal MSC phenotype, exhibited stable karyotype, had no mycoplasma contamination and had low endotoxin. These preliminary findings show that autologous expanded ADSC transplantation is a promising therapy for T2D treatment. This study is in continuation to monitor up to 12 months post transplantation- to record changes in the levels of blood glucose, C-peptide and HbA1C.
Abbreviations
ADSC: Adipose derived stem cells
aPRP: activated platelet rich plasma
DM: Diabetes mellitus
HbA1C: Hemoglobin A1c
MSCs: Mesenchymal stem cells
SVF: Stromal vascular fraction
T2D: Type 2 diabetes mellitus
Authors' Contribution
PTBL: prepared the patients, collected the adipose tissue, transplanted stem cells to the patients; PVP: collected the data, analysed the data, wrote the manuscript, suggested the treatment procedure; NBV, LTTD and NKP: cultured the stem cells, analysed the stem cells, prepared the stem cell products for transplantation.
Ethics approval
This study was approved by Ethical Comittee, Ministry of Health, Viet Nam.
References
-
B.
Antuna-Puente,
B.
Feve,
S.
Fellahi,
J.-P.
Bastard.
Adipokines: the missing link between insulin resistance and obesity. Diabetes & metabolism.
2008;
34
:
2-11
.
-
P.
Bhargava,
C.-H.
Lee.
Role and function of macrophages in the metabolic syndrome. Biochemical Journal.
2012;
442
:
253-262
.
-
M.
Burgos-Silva,
P.
Semedo-Kuriki,
C.
Donizetti-Oliveira,
P.B.
Costa,
M.A.
Cenedeze,
M.I.
Hiyane,
A.
Pacheco-Silva,
N.O.
Camara.
Adipose Tissue-Derived Stem Cells Reduce Acute and Chronic Kidney Damage in Mice. PLoS One.
2015;
10
:
e0142183
.
-
O.G.
Cantu-Rodriguez,
F.
Lavalle-Gonzalez,
M.A.
Herrera-Rojas,
J.C.
Jaime-Perez,
J.A.
Hawing-Zarate,
C.H.
Gutierrez-Aguirre,
C.
Mancias-Guerra,
O.
Gonzalez-Llano,
A.
Zapata-Garrido,
J.Z.
Villarreal-Perez.
Long-Term Insulin Independence in Type 1 Diabetes Mellitus Using a Simplified Autologous Stem Cell Transplant. J Clin Endocrinol Metab.
2016;
101
:
2141-2148
.
-
S.K.
Cheng,
E.Y.
Park,
A.
Pehar,
A.C.
Rooney,
G.I.
Gallicano.
Current progress of human trials using stem cell therapy as a treatment for diabetes mellitus. Am J Stem Cells.
2016;
5
:
74-86
.
-
K.S.
Cho,
H.J.
Roh.
Immunomodulatory effects of adipose-derived stem cells in airway allergic diseases. Curr Stem Cell Res Ther.
2010;
5
:
111-115
.
-
G.
Constantin,
S.
Marconi,
B.
Rossi,
S.
Angiari,
L.
Calderan,
E.
Anghileri,
B.
Gini,
S.D.
Bach,
M.
Martinello,
F.
Bifari.
Adipose-derived mesenchymal stem cells ameliorate chronic experimental autoimmune encephalomyelitis. Stem Cells.
2009;
27
:
2624-2635
.
-
J.
DeFuria,
A.C.
Belkina,
M.
Jagannathan-Bogdan,
J.
Snyder-Cappione,
J.D.
Carr,
Y.R.
Nersesova,
D.
Markham,
K.J.
Strissel,
A.A.
Watkins,
M.
Zhu.
B cells promote inflammation in obesity and type 2 diabetes through regulation of T-cell function and an inflammatory cytokine profile. Proceedings of the National Academy of Sciences.
2013;
110
:
5133-5138
.
-
S.
Devaraj,
M.R.
Dasu,
I.
Jialal.
Diabetes is a proinflammatory state: a translational perspective. Expert review of endocrinology & metabolism.
2010;
5
:
19-28
.
-
A.
El-Badawy,
N.
El-Badri.
Clinical Efficacy of Stem Cell Therapy for Diabetes Mellitus: A Meta-Analysis. PLoS One.
2016;
11
:
e0151938
.
-
A.U.
Engela,
M.J.
Hoogduijn,
K.
Boer,
N.H.
Litjens,
M.G.
Betjes,
W.
Weimar,
C.C.
Baan.
Human adipose-tissue derived mesenchymal stem cells induce functional de-novo regulatory T cells with methylated FOXP3 gene DNA. Clin Exp Immunol.
2013;
173
:
343-354
.
-
M.
Franquesa,
F.K.
Mensah,
R.
Huizinga,
T.
Strini,
L.
Boon,
E.
Lombardo,
O.
DelaRosa,
J.D.
Laman,
J.M.
Grinyo,
W.
Weimar.
Human adipose tissue-derived mesenchymal stem cells abrogate plasmablast formation and induce regulatory B cells independently of T helper cells. Stem Cells.
2015;
33
:
880-891
.
-
B.
Hirshberg.
Lessons learned from the international trial of the edmonton protocol for islet transplantation. Curr Diab Rep.
2007;
7
:
301-303
.
-
J.
Hu,
Y.
Wang,
H.
Gong,
C.
Yu,
C.
Guo,
F.
Wang,
S.
Yan,
H.
Xu.
Long term effect and safety of Wharton's jelly-derived mesenchymal stem cells on type 2 diabetes. Exp Ther Med.
2016;
12
:
1857-1866
.
-
R.
Jiang,
Z.
Han,
G.
Zhuo,
X.
Qu,
X.
Li,
X.
Wang,
Y.
Shao,
S.
Yang,
Z.C.
Han.
Transplantation of placenta-derived mesenchymal stem cells in type 2 diabetes: a pilot study. Front Med.
2011;
5
:
94-100
.
-
N.
Kamei,
K.
Tobe,
R.
Suzuki,
M.
Ohsugi,
T.
Watanabe,
N.
Kubota,
N.
Ohtsuka-Kowatari,
K.
Kumagai,
K.
Sakamoto,
M.
Kobayashi.
Overexpression of monocyte chemoattractant protein-1 in adipose tissues causes macrophage recruitment and insulin resistance. Journal of Biological Chemistry.
2006;
281
:
26602-26614
.
-
H.
Kanda,
S.
Tateya,
Y.
Tamori,
K.
Kotani,
K.-i.
Hiasa,
R.
Kitazawa,
S.
Kitazawa,
H.
Miyachi,
S.
Maeda,
K.
Egashira.
MCP-1 contributes to macrophage infiltration into adipose tissue, insulin resistance, and hepatic steatosis in obesity. The Journal of clinical investigation.
2006;
116
:
1494-1505
.
-
J.H.
Kim,
D.J.
Park,
J.C.
Yun,
M.H.
Jung,
H.D.
Yeo,
H.J.
Kim,
D.W.
Kim,
J.I.
Yang,
G.W.
Lee,
S.H.
Jeong.
Human adipose tissue-derived mesenchymal stem cells protect kidneys from cisplatin nephrotoxicity in rats. Am J Physiol Renal Physiol.
2012;
302
:
F1141-1150
.
-
Y.R.
Kuo,
C.C.
Chen,
S.
Goto,
I.T.
Lee,
C.W.
Huang,
C.C.
Tsai,
C.T.
Wang,
C.L.
Chen.
Modulation of immune response and T-cell regulation by donor adipose-derived stem cells in a rodent hind-limb allotransplant model. Plast Reconstr Surg.
2011;
128
:
661e-672e
.
-
J.
Liu,
A.
Divoux,
J.
Sun,
J.
Zhang,
K.
Clément,
J.N.
Glickman,
G.K.
Sukhova,
P.J.
Wolters,
J.
Du,
C.Z.
Gorgun.
Genetic deficiency and pharmacological stabilization of mast cells reduce diet-induced obesity and diabetes in mice. Nature medicine.
2009;
15
:
940-945
.
-
X.
Liu,
P.
Zheng,
X.
Wang,
G.
Dai,
H.
Cheng,
Z.
Zhang,
R.
Hua,
X.
Niu,
J.
Shi,
Y.
An.
A preliminary evaluation of efficacy and safety of Wharton's jelly mesenchymal stem cell transplantation in patients with type 2 diabetes mellitus. Stem Cell Res Ther.
2014;
5
:
57
.
-
M.
McCall,
A.M.
Shapiro.
Update on islet transplantation. Cold Spring Harb Perspect Med.
2012;
2
:
a007823
.
-
C.
Musilli,
S.
Paccosi,
L.
Pala,
G.
Gerlini,
F.
Ledda,
A.
Mugelli,
C.M.
Rotella,
A.
Parenti.
Characterization of circulating and monocyte-derived dendritic cells in obese and diabetic patients. Molecular immunology.
2011;
49
:
234-238
.
-
P.D.
Nguyen,
T.D.
Tran,
H.T.
Nguyen,
H.T.
Vu,
P.T.
Le,
N.L.
Phan,
N.B.
Vu,
N.K.
Phan,
P.
Van Pham.
Comparative Clinical Observation of Arthroscopic Microfracture in the Presence and Absence of a Stromal Vascular Fraction Injection for Osteoarthritis. Stem Cells Transl Med..
2016;
5
:
1-9
.
-
D.
Patsouris,
P.-P.
Li,
D.
Thapar,
J.
Chapman,
J.M.
Olefsky,
J.G.
Neels.
Ablation of CD11c-positive cells normalizes insulin sensitivity in obese insulin resistant animals. Cell metabolism.
2008;
8
:
301-309
.
-
A.
Rajwani,
R.
Cubbon,
S.
Wheatcroft.
Cell-specific insulin resistance: implications for atherosclerosis. Diabetes/metabolism research and reviews.
2012;
28
:
627-634
.
-
T.
Scully.
Diabetes in numbers. Nature.
2012;
485
:
S2-3
.
-
H.
Sell,
C.
Habich,
J.
Eckel.
Adaptive immunity in obesity and insulin resistance. Nature Reviews Endocrinology.
2012;
8
:
709-716
.
-
E.
Snarski,
A.
Milczarczyk,
K.
Halaburda,
T.
Torosian,
M.
Paluszewska,
E.
Urbanowska,
M.
Krol,
P.
Boguradzki,
K.
Jedynasty,
E.
Franek.
Immunoablation and autologous hematopoietic stem cell transplantation in the treatment of new-onset type 1 diabetes mellitus: long-term observations. Bone Marrow Transplant.
2016;
51
:
398-402
.
-
A.
Stepien,
N.L.
Dabrowska,
M.
Maciagowska,
R.P.
Macoch,
A.
Zolocinska,
S.
Mazur,
K.
Siennicka,
E.
Frankowska,
R.
Kidzinski,
M.
Chalimoniuk.
Clinical Application of Autologous Adipose Stem Cells in Patients with Multiple Sclerosis: Preliminary Results. Mediators Inflamm.
2016;
2016
:
5302120
.
-
S.
Talukdar,
G.
Bandyopadhyay,
D.
Li,
J.
Xu,
J.
McNelis,
M.
Lu,
P.
Li,
Q.
Yan,
Y.
Zhu,
J.
Ofrecio.
Neutrophils mediate insulin resistance in mice fed a high-fat diet through secreted elastase. Nature medicine.
2012;
18
:
1407-1412
.
-
Q.
Tong,
L.
Duan,
Z.
Xu,
H.
Wang,
X.
Wang,
Z.
Li,
W.
Zhang,
H.
Zheng.
Improved insulin secretion following intrapancreatic UCB transplantation in patients with T2DM. J Clin Endocrinol Metab.
2013;
98
:
E1501-1504
.
-
P.
Van Pham,
N.B.
Vu,
N.L.-C.
Phan,
D.M.
Le,
N.C.
Truong,
N.H.
Truong,
K.H.-T.
Bui,
N.K.
Phan.
Good manufacturing practice-compliant isolation and culture of human adipose derived stem cells. Biomedical Research and Therapy.
2014;
1
:
133-141
.
-
D.A.
Winer,
S.
Winer,
L.
Shen,
P.P.
Wadia,
J.
Yantha,
G.
Paltser,
H.
Tsui,
P.
Wu,
M.G.
Davidson,
M.N.
Alonso.
B cells promote insulin resistance through modulation of T cells and production of pathogenic IgG antibodies. Nature medicine.
2011;
17
:
610-617
.
-
D.
Wu,
A.B.
Molofsky,
H.-E.
Liang,
R.R.
Ricardo-Gonzalez,
H.A.
Jouihan,
J.K.
Bando,
A.
Chawla,
R.M.
Locksley.
Eosinophils sustain adipose alternatively activated macrophages associated with glucose homeostasis. Science.
2011;
332
:
243-247
.
-
H.
Xiang,
C.
Yang,
D.
Xiang Wu,
A.B.
Molofsky,
H.-E.
Liang,
R.R.
Ricardo-Gonzalez,
H.A.
Jouihan,
J.K.
Bando,
A.
Chawla,
R.M.
Locksley.
Eosinophils sustain adipose alternatively activated macrophages associated with glucose homeostasis. Science.
2011;
332
:
243-247
.
-
H.
Xiang,
C.
Yang,
T.
Xiang,
Z.
Wang,
X.
Ge,
F.
Li,
Y.
Su,
H.
Chen,
X.
Huang,
Q.
Zeng.
Residual beta-Cell Function Predicts Clinical Response After Autologous Hematopoietic Stem Cell Transplantation. Stem Cells Transl Med.
2016;
5
:
651-657
.
-
J.
Xv,
Q.
Ming,
X.
Wang,
W.
Zhang,
Z.
Li,
S.
Wang,
Y.
Li,
L.
Li.
Mesenchymal stem cells moderate immune response of type 1 diabetes. Cell Tissue Res. 10.1007/s00441-016-2499-2.
2016
.
Comments
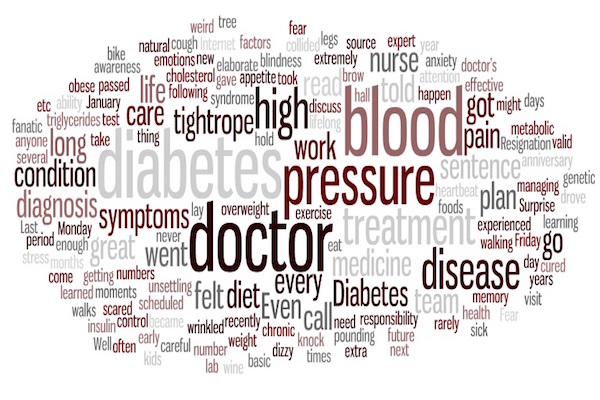
Downloads
Article Details
Volume & Issue : Vol 3 No 12 (2016)
Page No.: 1034-1044
Published on: 2016-12-15
Citations
Copyrights & License

This work is licensed under a Creative Commons Attribution 4.0 International License.
Search Panel
Pubmed
Google Scholar
Pubmed
Google Scholar
Pubmed
Google Scholar
Pubmed
Google Scholar
Pubmed
Search for this article in:
Google Scholar
Researchgate
- HTML viewed - 6472 times
- Download PDF downloaded - 1997 times
- View Article downloaded - 48 times