Direct reprogramming of fibroblasts into endothelial progenitor cells by defined factors
Abstract
Introduction: Endothelial progenitor cells (EPCs) are important progenitor cells in vasculogenesis as well as in tissue engineering. However, few EPCs can be isolated from bone marrow, peripheral blood and umbilical cord blood. Moreover, their in vitro proliferation potential is also limited. Therefore, this study aimed to produce EPCs from direct reprogramming of fibroblasts by transduction with certain specific factors. Methods: Human fibroblasts were collected from human skin by published protocols. The cells were transduced with 2 viral vectors containing 5 factors, including Oct3/4, Sox2, Klf4, c-Myc (plasmid 1), and VEGFR2 (plasmid 2). Transduced cells were treated with endothelial cell medium for 21 days. The cells were analyzed for expression of Oct3/4, Sox3, Klf4, c-Myc and VEGFR2 at day 5, and for EPC phenotype at day 21. Results: The results showed that after 5 days of transduction, fibroblasts acquired partial pluripotency. After 21 days of transduction and culture in endothelial cell medium, the cells exhibited endothelial markers (e.g. CD31 and VEGFR2) and formed blood vessel-like capillaries. Conclusion: Our findings suggest another strategy for direct reprogramming of fibroblasts into EPCs.
Introduction
Direct reprogramming is a process wherein a differentiated adult cell converts into another differentiated somatic cell. As a result, direct conversion does not pass through an undifferentiated pluripotent stage Van Pham, 2015. This strategy enables the generation of patient-specific cell types, without formation of pluripotent stem cell-induced tumors prior to differentiation. Therefore, this reprogramming technology has been a potent tool for regenerative medicine.
Endothelial progenitor cells (EPCs) are one of the most important progenitor cells in tissue engineering, especially in vasculogenesis and angiogenesis Chong et al., 2016. The use of EPCs has provided effective results in treating hindlimb ischemia Flex et al., 2016Yu et al., 2009, stroke Bai et al., 2015Li et al., 2015, diabetic ulcer Gallagher et al., 2006, and myocardial infarction Kawamoto et al., 2003. Although EPCs have many advantages, one major disadvantage is that very low numbers of these cells can be isolated from bone marrow Hristov et al., 2003, peripheral blood Donndorf et al., 2015, and umbilical cord blood Schmidt et al., 2004Van Phuc et al.,2012. Moreover, their proliferation potential is limited. Therefore, this study investigated the production of EPCs from direct reprogramming of fibroblasts via transduction with defined factors. We show that fibroblasts were, indeed, differentiated into EPCs by endothelial cell medium containing specific endothelial differentiation factors. Thus, the study herein demonstrates the application of a direct reprogramming technology to reprogram fibroblasts into partial pluripotent stem cells.
Methods
Isolation and culture of human fibroblasts and HEK 293T
Human fibroblasts (HFs) were isolated from foreskin, according to a previously published protocol Van Pham et al., 2016. Cells were cultured in DMEM/F12 complete (DMEM/F12, 10% FBS and 1% antibiotic; all reagents were bought from Life Technologies, Carlsbad, CA) until cells reached 80-90% confluency on surface flask. The HFs were then sub-cultured to the 3rd passage. Cells were cryopreserved in liquid nitrogen until use in experiments. HEK 293T cells were obtained from a commercial source (Life Technologies, Carlsbad, CA). The cells were thawed and cultured at 5 x 106 cells in a 25cm2 flask. HEK 293T were also cultured in DMEM/F12 complete.
Viral vector production
There were 2 viral vectors used in this study. Vector 1 contained four factors: Oct3/4, Sox2, Klf4 and c-Myc (OKSIM); Vector 2 contained VEGFR2. HEK 293T were trypsinized to collect single cells. Then, a plasmid containing c-Myc (OKSIM) was cotransfected into HEK293T cells with pCMV-VSV-GRSV- Rev and pCMV-dR8.2 (Addgene, Cambridge, MA) to produce Vector 1. Similarly, Vector 2 was produced by transfection of HEK293T cells with plasmids expressing VEGFR2, pCMV-VSV-G-RSVRev, and pCMV-dR8.2. The mix was transferred into 2 mm electroporation cuvettes and transfected into cells. Immediately after, pre-warmed medium was gently added to the transfection mix and transferred into 6-well plates. The plates was incubated at 37oC, 5% CO2 for 24 h. After 36 h, supernatant was collected to extract viral particles by centrifugation.
Transduction into HFs
On the day of transduction, HFs were treated with polyprene at the final concentration of 8 μg/mL in 6 h, then transduced with both viral vector 1 (containing Oct3/4, Sox2, Klf4, and c-Myc) and viral vector 2 (containing VEGFR2 only). Transduction was repeated two independent times, without polyprene treatment the second time. The medium was refreshed after 2 d of transduction by endothelial cell medium and every 3 d until day 21. The endothelial cell medium was prepared by M200 medium supplemented with 2% fetal bovine serum (FBS), 10 ng/mL vascular endothelial growth factor (VEGF), 5 ng/mL epithelial growth factor (EGF), 5 ng/mL basic fibroblast growth factor (bFGF), 1 μg/ml hydrocortisone, and 90 μg/mL heparin (all chemicals and media were bought from Life Technologies, Carlsbad, CA).
Gene expression by reverse transcription polymerase chain reaction (RT-PCR)
Total cellular RNA was extracted with the use of easy- BLUETM Total RNA Extraction Kit (iNtRON, Korea) from cells at day 5. Inducible EPC (iEPC) gene expression was detected using a one-step RT-PCR premix kit (iNtRON, Korea), according to the manufacturer’s protocol. The reaction was performed in a thermal realtime PCR cycler (Eppendorf, Germany) with amplification of over 30 cycles at 94°C for 20 s (denaturing), 50-60°C for 10 s (annealing), and 72°C for 30 min (primer extension). The primers (AIT Biotech, Singapore) used in this experiment are listed in Table 1 (F: forward, R: reverse). RNA for GAPDH was co-amplified to assess the quality of the samples.
Immunocytochemistry
HFs were seeded in 48-well plates the day before the this experiment. The medium was removed and washed twice with PBS. The cells were then fixed with 4% paraformaldehyde for 30 minutes and washed twice with PBS. Cells were stained with anti-CD31 monoclonal antibody conjugated with PE (Santa Cruz Biotechnology, Santa Cruz, CA) for 30 min, then counterstained with Hoechst 33342 for 15 min to visualize the nuclei. Cells were washed twice with PBS and observed under a fluorescent microscope (Carl-Zeiss, Oberkochen, Germany).
Capillary-like structure formation assay
Cells (2 x 104) were seeded on 96-well flat-bottom plates coated with 30 μL Matrigel (Lifetechnologies) and cultured in EGM-2 medium. Eighteen hours after incubation, capillary-like structures were observed under an Axiovert microscope (Carl-Zeiss, Oberkochen, Germany).
Statistical analysis
Statistical analyses of all endpoints were performed using the two-sided Student’s t test or one-way analysis of variance. All data are presented as mean ± SD. p < 0.05 was considered statistically significant. Data were analyzed with Prism 6.0 software.
Results
Culture and proliferation of human fibroblasts and HEK293T cells
Dermal foreskin fragments were cultured for 21 d to isolate dermal fibroblasts. Under microscopy, fibroblasts could be seen migrating from the tissue fragments at day 5. From day 15, cells began to rapidly proliferate ( Figure 1A ). The cells became homogenous after 3 passages of sub-culturing ( Figure 1B ). The hemogenicity of the cell population was confirmed by CD90 surface expression; flow cytometry analysis of CD90 expression showed that 100% fibroblasts expressed the gene ( Figure 1C ).
HEK293T cells were thawed from the commercial kit, and then cultured in standard conditions, according to the manufacturer’s guidelines. After thawing, the cells were cultured until they reached confluency (typically after 5 d). All cells were sub-cultured at least 2 times before use in further studies ( Figure 1D ).
Transduced fibroblasts were enhanced to express pluripotent genes
After transduction with 2 viral vectors for 7 d, transduced fibroblasts were evaluated for expression of Oct4, Sox2, cMyc and Klf4. The expression of these factors were vital for triggering the epigenetic reprogramming. The RT-PCR results showed that after 7 d of transduction, fibroblasts showed increased expression of the epigenetic reprogramming markers, i.e. Oct4, Sox2, cMyc and Klf4 ( Figure 2 ).
Changes in cell shape during mesenchymal to endothelial transition
After transducing with 2 retroviral vectors containing 5 factors, the fibroblasts were cultured in the endothelial cell medium. This medium was derived from endothelial cells cultured with 2% FBS(to inhibit growth of non-transduced fibroblasts) and supplemented with hydrocortisone (to stimulate the growth of endothelial cells). Under this condition, the majority of cells died after 48-72h of treatment ( Figure 3A ). Some surviving cells formed clusters of cells ( Figure 3B ). From day 5 (after culture in endothelial cell medium), surviving cells clearly changed their cell shape. Almost all cells converted from a spindle shape to a round shape (similar to that of HUVECs) ( Figure 3C ). The cells gradually became a homogenous cell population after 21 d of culture( Figure 3D ).
Cell size cell population
Besides the change in shape that was observed under microscopy, the transduced cells also changed in cell size. The size of the cells before and after transduction with viral vectors containing 5 target genes were analyzed by flow cytometry and are presented at Figure 4 . After transduction with viral vectors containing the target genes and cultured in endothelial cell medium, the fibroblast size significantly changed. From flow cytometry data, a small population with higher forward scatter (FSC) and the same side scatter (SSC) became visible ( Figure 4 ). The cell size was larger than that of fibroblasts.
Upregulation of CD31 and VEGFR2
Expression of CD31 and VEGFR2 were determined by flow cytometry and immunocytochemistry. The percentage of CD31 positive cells was 3.48 ±0.92 % (p>0.05) in transduced cells, and 0% in the control. There was a significant increase in the percentage of VEGFR2 positive cells too; the percentage was 91.84 ± 6.41% in transduced cells, and 43.07 ± 3.43% in the control.
The expression of CD31 was also evaluated but via immunocytochemistry with anti-CD31 monoclonal antibody-PE. The results are represented in Figure 5 . The results showed that fibroblasts did not express CD31 yet the transduced cells did express the CD31 marker ( Figure 5 ).
In vitro formation of blood vessel-like capillaries
Transduced fibroblasts were cultured in culture medium for 20 d. They were used to evaluate the invitro formation of blood vessel like capillary in matrigel. The results showed that after 18 h of incubation, transduced cells were capable of forming capillary structure similar to blood vessels ( Figure 6 ).Results are similar to using HUVECs; results suggest that transduced cells cultured in endothelial cell medium can exhibit endothelial progenitor cell bio-activity in vitro.
Discussion
Endothelial progenitor cells as well as endothelial cells are important cell sources for regenerative medicine and tissue engineering. Therefore, this study aimed at generating EPCs by direct reprogramming of fibroblasts with 5 factors, including Oct3/4, Sox-2, Klf4, c-Myc and VEGFR2, in combination with culture in endothelial cell medium. Our study showed that the above conditions induced the generation of functional EPCs.
Fibroblasts were successfully isolated from foreskin. This cell population was a homogenous population with 100% CD90-expressing cells. Indeed, CD90 expression is considered a fibroblast marker Kisselbach et al., 2009. Fibroblasts are common cells in the human body, and are especially easily to isolate and are very proliferative. Therefore, fibroblasts are the predominant cells used in the study as well as in applications of epigenetic reprogramming, including direct reprogramming. Indeed, fibroblasts are used to reprogram and induce pluripotent stem cells Alawad et al.,2016Takahashi and Yamanaka, 2006Yu et al., 2007, myoblasts Choi et al., 1990Davis et al., 1987Lassar et al., 1989, adipocytes Tontonoz et al., 1994, macrophages Feng et al., 2008, cardiomyocytes Efe et al., 2011, neuron Yoo et al.,2011, myocytes Bichsel et al., 2013, and endothelial progenitor cells Li et al., 2013Van Pham et al., 2016Wong and Cooke, 2016. In this study, we used fibroblasts to directly induce reprogramming into EPCs.
To date, there have been various approaches used different induction factors to direct reprogramming of fibroblasts into EPCs. For example, in the first study involving direct reprogramming of fibroblasts to EPCs, Margariti et al. used 4 factors, including OCT4, SOX2, KLF4, and c-MYC to induce fibroblasts for 4 days. Then, differentiation of particle induced pluripotent stem cells into endothelial cells was done by treatment with defined media and culture conditions Margariti et al., 2012. Subsequently, Li et al investigated the removal of the Sox-2 gene and two other genes (Oct4 and Klf4), in combination with soluble factors Li et al., 2013. Since the efficacy of these procedures was extremely low, Han et al. 2014 tried a combination of Foxo1, Er71, Klf2, Tal1, and Lmo2Han et al., 2014. Recently, another factor for use as a single factor for direct reprogramming of fibroblasts to EPCs was suggested Lee et al., 2014Morita et al.,2015; single factor ETV2 was capable of inducing fibroblasts to acquire EPC phenotype. In 2016, the efficacy of this procedure was increased by combining ETV2 transfection with hypoxia Van Pham et al., 2016.
In this study, we investigated a strategy similar to the one by Margariti et al. Margariti et al., 2012. Initially, fibroblasts are induced to acquire partial pluripotency using 4 factors expressed in the first viral vectors (Oct3/4, Sox-2, Klf4 and c-Myc). Indeed, these factors could successfully reprogram many adult cells toward pluripotent stem cells. To improve on this strategy, we used another vector containing VEGFR2, a receptor for VEGF that has many roles. VEGF is critical signaling protein involved in both vasculogenesis and angiogenesis Shibuya and Claesson-Welsh, 2006, and it serves as a mitogen for endothelial cells Hoeben et al., 2004. It can also stimulate the migration of endothelial cells. Upregulation of VEGFR2 on fibroblasts enhances the effects of VEGF on both mitogenesis and migration.
Thus, we investigated if partial pluripotent stem cells could be directly induced into EPCs by endothelial cell medium. The endothelial cell medium contains critical factors to direct induction of partial pluripotent stem cells into EPCs. For example, the medium contains low fetal bovine serum (2% as opposed to 10% used for fibroblasts), VEGF, hydrocortisone, and heparin. The low serum medium is not only suitable for endothelial cells but also can inhibit the proliferation of fibroblasts, particularly non-transduced fibroblasts. Hydrocortisone is an important factor in the endothelial cell medium, with an important role of triggering endothelial cell differentiation, while inhibiting mesenchymal characteristics Furihata et al., 2015. Heparin also has an essential role in endothelial cell growth; it is considered important in modulating the availability and stability of potent growth-regulating agents for endothelial cells D’Amore, 1990. Moreover, heparin also protects vascular endothelial cells from injury induced by TNFα and sepsis; the protective mechanisms are related to effects of heparin on the histone methylation of promoter region and the regulation of heparin on the MAPK and NF-κB signal pathways Ma and Bai, 2015. Heparin also binds to angiogenic growth factors and some pro-angiogenic receptors, as well as to angiogenic inhibitors which regulate angiogenesis Chiodelli et al., 2015. Taken together, all the above factors can drive partialpluripotent stem cells toward to EPCs. The process and mechanism of direct reprogramming of fibroblasts into EPCs are proposed in Figure 7 .
Importantly, results from our study show that the process of reprogramming occurs and that it is feasible to produce functional endothelial progenitor cells. Although the efficacy of this process was low, a small population with markers of endothelial progenitor cells (CD31+VEGFR2+) could be produced. Moreover, these cells could perform in vitrofunctions of EPCs, such as blood vessel-like capillary formation. The properties of our EPCs are similar to ones of previously published studies Lee et al., 2014Morita et al.,2015Van Pham et al., 2016.
Conclusion
EPCs can participate in both vasculogenesis and angiogenesis. Therefore, they can be used in treatment of ischemia-related diseases or blood vessel regeneration. However, the percentage of EPCs in blood and other tissues is extremely low. Our study demonstrated that EPCs could be feasibly produced by direct reprogramming of fibroblasts. Five factors were necessary in the cell medium: Oct3/4, Sox-2, Klf4, c-Myc and VRGFR2. Additionally, endothelial cell medium (containing low fetal bovine serum, hydrocortisone and heparin) was also critical for the induction. The EPCs expressed CD31 and VEGFR2, and formed blood vessel-like capillaries in vitro. These results suggest another strategy to directly reprogram fibroblasts into EPCs for potential use in regenerative medicine.
References
-
A.
Alawad,
O.
Alhazzaa,
S.
Altuwaijri,
M.
Alkhrayef,
F.
Alagrafi,
Z.
Alhamdan,
A.
Alenazi,
S.
Alharbi,
M.
Hammad.
Generation of human iPS cell line SKiPSc1 from healthy Human Neonatal Foreskin Fibroblast cells. Stem Cell Res.
2016;
17
:
158-160
.
-
Y.Y.
Bai,
X.G.
Peng,
L.S.
Wang,
Z.H.
Li,
Y.C.
Wang,
C.Q.
Lu,
J.
Ding,
P.C.
Li,
Z.
Zhao,
S.H.
Ju.
Bone Marrow Endothelial Progenitor Cell Transplantation After Ischemic Stroke: An Investigation Into Its Possible Mechanism. CNS Neurosci Ther.
2015;
21
:
877-886
.
-
C.
Bichsel,
D.
Neeld,
T.
Hamazaki,
L.J.
Chang,
L.J.
Yang,
N.
Terada,
S.
Jin.
Direct reprogramming of fibroblasts to myocytes via bacterial injection of MyoD protein. Cell Reprogram.
2013;
15
:
117-125
.
-
P.
Chiodelli,
A.
Bugatti,
C.
Urbinati,
M.
Rusnati.
Heparin/Heparan sulfate proteoglycans glycomic interactome in angiogenesis: biological implications and therapeutical use. Molecules.
2015;
20
:
6342-6388
.
-
J.
Choi,
M.L.
Costa,
C.S.
Mermelstein,
C.
Chagas,
S.
Holtzer,
H.
Holtzer.
MyoD converts primary dermal fibroblasts, chondroblasts, smooth muscle, and retinal pigmented epithelial cells into striated mononucleated myoblasts and multinucleated myotubes. Proc Natl Acad Sci U S A.
1990;
87
:
7988-7992
.
-
M.S.
Chong,
W.K.
Ng,
J.K.
Chan.
Concise Review: Endothelial Progenitor Cells in Regenerative Medicine: Applications and Challenges. Stem Cells Transl Med.
2016;
5
:
530-538
.
-
P.A.
D’Amore.
Heparin-endothelial cell interactions. Haemostasis 20 Suppl.
1990;
1
:
159-165
.
-
R.L.
Davis,
H.
Weintraub,
A.B.
Lassar.
Expression of a single transfected cDNA converts fibroblasts to myoblasts. Cell.
1987;
51
:
987-1000
.
-
P.
Donndorf,
L.
Lube,
C.
Lux,
A.
Skorska,
G.
Steinhoff,
K.
Kraft.
Mobilization of Bone Marrow-Derived Endothelial Progenitor Cells following Finnish Sauna: A Pilot Study. Forsch Komplementmed.
2015;
22
:
246-250
.
-
J.A.
Efe,
S.
Hilcove,
J.
Kim,
H.
Zhou,
K.
Ouyang,
G.
Wang,
J.
Chen,
S.
Ding.
Conversion of mouse fibroblasts into cardiomyocytes using a direct reprogramming strategy. Nat Cell Biol.
2011;
13
:
215-222
.
-
R.
Feng,
S.C.
Desbordes,
H.
Xie,
E.S.
Tillo,
F.
Pixley,
E.R.
Stanley,
T.
Graf.
PU.1 and C/EBPalpha/beta convert fibroblasts into macrophage-like cells. Proc Natl Acad Sci U S A.
2008;
105
:
6057-6062
.
-
A.
Flex,
F.
Biscetti,
M.G.
Iachininoto,
E.R.
Nuzzolo,
N.
Orlando,
S.
Capodimonti,
F.
Angelini,
C.G.
Valentini,
M.
Bianchi,
L.M.
Larocca.
Human cord blood endothelial progenitors promote post-ischemic angiogenesis in immunocompetent mouse model. Thromb Res.
2016;
141
:
106-111
.
-
T.
Furihata,
S.
Kawamatsu,
R.
Ito,
K.
Saito,
S.
Suzuki,
S.
Kishida,
Y.
Saito,
A.
Kamiichi,
K.
Chiba.
Hydrocortisone enhances the barrier properties of HBMEC/ciβ, a brain microvascular endothelial cell line, through mesenchymal-toendothelial transition-like effects. Fluids and Barriers of the CNS.
2015;
12
:
7
.
-
K.A.
Gallagher,
L.J.
Goldstein,
S.R.
Thom,
O.C.
Velazquez.
Hyperbaric oxygen and bone marrow-derived endothelial progenitor cells in diabetic wound healing. ascular.
2006;
14
:
328-337
.
-
J.K.
Han,
S.H.
Chang,
H.J.
Cho,
S.B.
Choi,
H.S.
Ahn,
J.
Lee,
H.
Jeong,
S.W.
Youn,
H.J.
Lee,
Y.W.
Kwon.
Direct conversion of adult skin fibroblasts to endothelial cells by defined factors. Circulation.
2014;
130
:
1168-1178
.
-
A.
Hoeben,
B.
Landuyt,
M.S.
Highley,
H.
Wildiers,
A.T.
Van Oosterom,
E.A.
De Bruijn.
Vascular endothelial growth factor and angiogenesis. Pharmacol Rev.
2004;
56
:
549-580
.
-
M.
Hristov,
W.
Erl,
P.C.
Weber.
Endothelial progenitor cells: isolation and characterization. Trends in cardiovascular medicine.
2003;
13
:
201-206
.
-
A.
Kawamoto,
T.
Tkebuchava,
J.
Yamaguchi,
H.
Nishimura,
Y.S.
Yoon,
C.
Milliken,
S.
Uchida,
O.
Masuo,
H.
Iwaguro,
H.
Ma.
Intramyocardial transplantation of autologous endothelial progenitor cells for therapeutic neovascularization of myocardial ischemia. Circulation.
2003;
107
:
461-468
.
-
L.
Kisselbach,
M.
Merges,
A.
Bossie,
A.
Boyd.
CD90 Expression on human primary cells and elimination of contaminating fibroblasts from cell cultures. ytotechnology.
2009;
59
:
31-44
.
-
A.B.
Lassar,
M.J.
Thayer,
R.W.
Overell,
H.
Weintraub.
Transformation by activated ras or fos prevents myogenesis by inhibiting expression of MyoD1. Cell.
1989;
58
:
659-667
.
-
S.
Lee,
C.
Park,
J.W.
Han,
J.Y.
Kim,
K.
Cho,
E.J.
Kim,
S.
Kim,
S.-J.
Lee,
H.J.
An,
M.Y.
Sin.
Direct Reprogramming of Human Dermal Fibroblasts into Endothelial Cells Using a Single Transcription Factor. Circulation.
2014;
130
:
A18205-A18205
.
-
J.
Li,
N.F.
Huang,
J.
Zou,
T.J.
Laurent,
J.C.
Lee,
J.
Okogbaa,
J.P.
Cooke,
S.
Ding.
Conversion of human fibroblasts to functional endothelial cells by defined factors. Arterioscler Thromb Vasc Biol.
2013;
33
:
1366-1375
.
-
S.H.
Li,
D.D.
Wang,
Y.J.
Xu,
G.D.
Ma,
X.Y.
Li,
W.J.
Liang.
Exogenous hTERT gene transfected endothelial progenitor cells from bone marrow promoted angiogenesis in ischemic myocardium of rats. Int J Clin Exp Med.
2015;
8
:
14447-14453
.
-
J.
Ma,
J.
Bai.
Protective effects of heparin on endothelial cells in sepsis. Int J Clin Exp Med.
2015;
8
:
5547-5552
.
-
A.
Margariti,
B.
Winkler,
E.
Karamariti,
A.
Zampetaki,
T.N.
Tsai,
D.
Baban,
J.
Ragoussis,
Y.
Huang,
J.D.
Han,
L.
Zeng.
Direct reprogramming of fibroblasts into endothelial cells capable of angiogenesis and reendothelialization in tissueengineered vessels. Proc Natl Acad Sci U S A.
2012;
109
:
13793-13798
.
-
R.
Morita,
M.
Suzuki,
H.
Kasahara,
N.
Shimizu,
T.
Shichita,
T.
Sekiya,
A.
Kimura,
K.
Sasaki,
H.
Yasukawa,
A.
Yoshimura.
ETS transcription factor ETV2 directly converts human fibroblasts into functional endothelial cells. Proc Natl Acad Sci U S A.
2015;
112
:
160-165
.
-
D.
Schmidt,
C.
Breymann,
A.
Weber,
C.I.
Guenter,
S.
Neuenschwander,
G.
Zund,
M.
Turina,
S.P.
Hoerstrup.
Umbilical cord blood derived endothelial progenitor cells for tissue engineering of vascular grafts. The Annals of thoracic.
2004;
surgery78
:
2094-2098
.
-
M.
Shibuya,
L.
Claesson-Welsh.
Signal transduction by VEGF receptors in regulation of angiogenesis and lymphangiogenesis. Exp Cell Res.
2006;
312
:
549-560
.
-
K.
Takahashi,
S.
Yamanaka.
Induction of pluripotent stem cells from mouse embryonic and adult fibroblast cultures by defined factors. Cell.
2006;
126
:
663-676
.
-
P.
Tontonoz,
E.
Hu,
B.M.
Spiegelman.
Stimulation of adipogenesis in fibroblasts by PPAR gamma 2, a lipid-activated transcription factor. Cell.
1994;
79
:
1147-1156
.
-
P.
Van Pham.
Direct reprogramming of somatic cells: an update. Biomedical Research and Therapy.
2015;
2
:
1-10
.
-
P.
Van Pham,
N.B.
Vu,
H.T.
Nguyen,
O.T.
Huynh,
M.T.
Truong.
Significant improvement of direct reprogramming efficacy of fibroblasts into progenitor endothelial cells by ETV2 and hypoxia. Stem Cell Res Ther.
2016;
7
:
10-4
.
-
P.
Van Phuc,
V.B.
Ngoc,
D.H.
Lam,
N.T.
Tam,
P.Q.
Viet,
P.K.
Ngoc.
Isolation of three important types of stem cells from the same samples of banked umbilical cord blood. Cell and tissue banking.
2012;
13
:
341-351
.
-
W.T.
Wong,
J.P.
Cooke.
Therapeutic transdifferentiation of human fibroblasts into endothelial cells using forced expression of lineage-specific transcription factors. J Tissue Eng.
2016;
7
:
2041731416628329
.
-
A.S.
Yoo,
A.X.
Sun,
L.
Li,
A.
Shcheglovitov,
T.
Portmann,
Y.
Li,
C.
Lee-Messer,
R.E.
Dolmetsch,
R.W.
Tsien,
G.R.
Crabtree.
MicroRNA-mediated conversion of human fibroblasts to neurons. Nature.
2011;
476
:
228-231
.
-
J.
Yu,
M.A.
Vodyanik,
K.
Smuga-Otto,
J.
Antosiewicz-Bourget,
J.L.
Frane,
S.
Tian,
J.
Nie,
G.A.
Jonsdottir,
V.
Ruotti,
R.
Stewart.
Induced pluripotent stem cell lines derived from human somatic cells. Science.
2007;
318
:
1917-1920
.
-
J.X.
Yu,
X.F.
Huang,
W.M.
Lv,
C.S.
Ye,
X.Z.
Peng,
H.
Zhang,
L.B.
Xiao,
S.M.
Wang.
Combination of stromalderived factor-1alpha and vascular endothelial growth factor genemodified endothelial progenitor cells is more effective for ischemic neovascularization. J Vasc Surg.
2009;
50
:
608-616
.
Comments
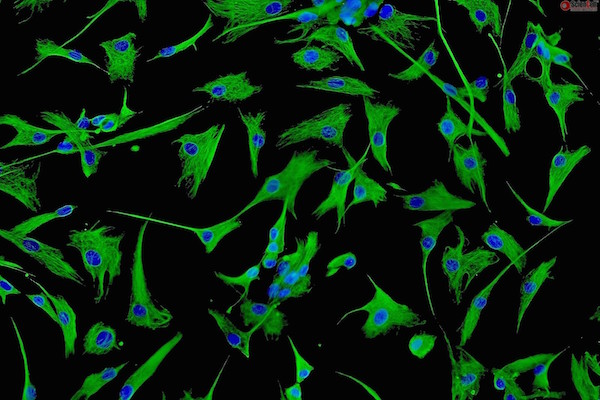
Downloads
Article Details
Volume & Issue : Vol 3 No 08 (2016)
Page No.: 780-789
Published on: 2016-08-30
Citations
Copyrights & License

This work is licensed under a Creative Commons Attribution 4.0 International License.
Search Panel
Pubmed
Google Scholar
Pubmed
Google Scholar
Pubmed
Google Scholar
Pubmed
Search for this article in:
Google Scholar
Researchgate
- HTML viewed - 5487 times
- Download PDF downloaded - 1323 times
- View Article downloaded - 12 times